Molecular motors, the minuscule engines within our cells, play pivotal roles far beyond their diminutive size. These remarkable biological machines convert chemical energy into mechanical work, powering processes essential for life. From transporting cellular cargo to facilitating muscle contractions, molecular motors work tirelessly behind the scenes of biological systems.
The primary distinction between linear and rotary molecular motors lies in their mode of action. Linear motors move along a path in a straight line, similar to a train on tracks, while rotary motors rotate around a fixed axis, akin to the way a windmill spins. Each type utilizes energy to perform work, but they do so in uniquely different ways that are crucial for various biological functions and technological applications.
Exploring the differences between linear and rotary molecular motors reveals a world of precision engineering at the molecular level. These motors are not only fundamental to understanding how life operates on a microscopic scale but also hold the key to advancing nanotechnology. By studying their mechanisms, scientists and engineers can harness their power for innovative medical treatments and the development of nanoscale machinery.
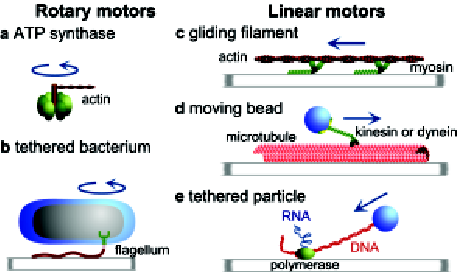
Basics of Molecular Motors
What Are Molecular Motors?
Molecular motors are proteins that transform chemical energy into mechanical force, enabling movement and various activities within living cells. These motors are the workhorses behind many critical cellular functions, from muscle contraction and cell division to the transport of molecules and organelles within the cell.
Types of Molecular Motors
Molecular motors can be broadly categorized into two types: linear and rotary. Linear motors move along a path in a straight line, while rotary motors spin around a fixed axis. This distinction is crucial for their specific roles and applications in biological systems and technology.
Linear Molecular Motors
Definition and Mechanism
Linear molecular motors operate by moving along a linear track, such as microtubules or actin filaments, within the cell. They convert the energy from ATP hydrolysis into mechanical work, allowing them to “walk” along these tracks and transport cellular cargo.
How Linear Motors Work
- Binding: The motor protein binds to a microtubule or actin filament.
- Power Stroke: ATP hydrolysis provides energy for the motor to take a step along the filament.
- Release and Reset: The motor releases the spent ADP and binds a new ATP, preparing for the next cycle.
Examples in Nature
Kinesin and Dynein
- Kinesin moves cargo towards the plus end of microtubules, generally outward from the cell center.
- Dynein, in contrast, moves towards the minus end of microtubules, pulling cargo towards the cell center.
These motors are essential for processes such as mitosis, meiosis, and neuronal transport.
Applications
Linear molecular motors have applications in both medicine and technology. In medicine, they are being explored for targeted drug delivery systems. Technologically, they inspire the development of nanoscale transport systems, mimicking the cargo delivery system of the cell.
Rotary Molecular Motors
Definition and Mechanism
Rotary molecular motors rotate around an axis to perform their function. This rotation can convert chemical energy directly into rotational force, which can be used for various tasks, including ATP synthesis and bacterial locomotion.
How Rotary Motors Operate
The mechanism of rotary motors involves the conversion of energy from ATP or an ion gradient into rotational motion. This motion can be continuous or step-wise, depending on the motor’s structure and the energy source.
Examples in Nature
ATP Synthase and Bacterial Flagella
- ATP synthase is a rotary motor that synthesizes ATP, the cell’s energy currency, by using the proton gradient across the mitochondrial membrane.
- Bacterial flagella utilize a rotary motor mechanism to propel bacteria through their environment, crucial for mobility and survival.
These examples highlight the diversity and importance of rotary motors in nature.
Applications
Energy Production and Nanotechnology
Rotary molecular motors are pivotal in energy production within cells and have inspired applications in nanotechnology. For instance, designing synthetic motors that mimic ATP synthase could lead to new ways of energy generation on a nanoscale. Moreover, understanding bacterial flagellar motors can inform the development of micro-robots or nanoscale propellers for medical applications, such as targeted therapy delivery.
Key Differences Between Linear and Rotary Molecular Motors
Structural Variations
Physical Structure Comparison
Linear and rotary molecular motors differ significantly in their physical structures, reflecting their distinct functions. Linear motors, such as kinesin and dynein, are elongated proteins that bind and “walk” along cellular structures like microtubules. Their structure allows for straight-line movements across significant distances within the cell. Each step they take is a result of conformational changes within the motor’s protein structure, facilitated by ATP hydrolysis.
In contrast, rotary motors like ATP synthase and the bacterial flagellar motor possess a circular architecture. This design enables them to rotate around an axis. ATP synthase, for example, has a rotor and stator parts, allowing it to convert the energy from a proton gradient into the rotational force necessary for ATP production. The bacterial flagellar motor rotates in a similar fashion, propelling bacteria through their environment.
Functional Contrast
How Their Movements Differ
The movement of linear and rotary molecular motors is fundamentally different. Linear motors translate chemical energy into linear motion, moving in a directed manner along a predetermined path. This capability is essential for transporting cellular cargo to specific locations within the cell.
Rotary motors, however, transform energy into rotational motion. This rotation can be harnessed for a variety of tasks, from synthesizing ATP in the case of ATP synthase to driving the locomotion of bacteria via their flagella. The rotational movement of these motors is continuous or can occur in discrete steps, depending on the energy source and specific motor type.
Energy Consumption
Differences in Energy Use
Another key difference between linear and rotary molecular motors lies in their energy consumption patterns. Linear motors consume ATP with each “step” they take along their path, with the rate of ATP hydrolysis directly tied to their speed and the load they are transporting. This consumption is critical for their ability to perform work over distances.
Rotary motors, on the other hand, may operate under different energy consumption models. For instance, ATP synthase uses the proton gradient across the mitochondrial membrane as its primary energy source, converting this potential energy into the mechanical energy of rotation, which is then used to synthesize ATP. The bacterial flagellar motor utilizes the flow of ions across the bacterial membrane to drive its rotation, showcasing a unique model of energy use.
Significance in Research and Technology
Biomedical Implications
Drug Delivery and Diagnostics
The understanding of molecular motors has profound implications for biomedicine, especially in the areas of drug delivery and diagnostics. Linear motors, with their ability to navigate the intricate pathways of the cellular environment, inspire the design of targeted drug delivery systems. These systems aim to mimic the motors’ targeted transport capabilities, delivering therapeutic agents directly to specific cells or tissues, thereby increasing efficacy and reducing side effects.
Furthermore, the study of molecular motors contributes to the development of advanced diagnostic tools. For example, technologies that replicate the motility of molecular motors can potentially be used to navigate and map the human body at the cellular level, offering unprecedented precision in identifying and diagnosing diseases.
Technological Innovations
Nanomachines and Robotics
The principles underlying the operation of molecular motors are also being harnessed to drive advancements in nanotechnology and robotics. Nanomachines, designed to perform specific tasks at the molecular level, often take inspiration from the mechanisms of molecular motors. By replicating the linear motion of kinesin or the rotational movement of ATP synthase, engineers are developing nanoscale devices capable of performing tasks ranging from material assembly to sensing in ways that were previously unimaginable.
Robotic applications, particularly those focusing on micro- and nanoscale operations, also benefit from insights gained from the study of molecular motors. The development of miniature robots that can navigate complex environments, conduct repairs at the microscopic level, or even assemble microscopic structures, owes much to the understanding of how molecular motors convert chemical energy into mechanical work.
Future Directions
Research Trends
Current Studies on Molecular Motors
Current research on molecular motors focuses on understanding their structure and mechanics at an even deeper level, leveraging advancements in cryo-electron microscopy and computational modeling. Scientists are exploring the intricate details of how these motors convert energy into motion, seeking to uncover new principles that can be applied in biotechnology and materials science.
Studies are also aimed at synthesizing artificial molecular motors, with the goal of achieving controlled motion in synthetic systems. This research holds the promise of creating entirely new classes of molecular machines, capable of performing complex tasks in industrial, medical, and environmental applications.
Potential Applications
Future Uses in Technology and Medicine
The potential applications of molecular motors extend across various fields, promising revolutionary advances in technology and medicine. In the medical realm, the future may see nano-robots powered by principles derived from molecular motors, capable of performing surgeries at the cellular level or precisely delivering drugs to combat diseases at their source.
In technology, molecular motors may lead to the development of new materials with dynamic properties, including the ability to self-heal, change shape in response to environmental conditions, or even assemble spontaneously into complex structures. Additionally, the principles of energy conversion and mechanical work observed in molecular motors are inspiring sustainable energy solutions, mimicking nature’s efficiency at harvesting and utilizing energy.
Frequently Asked Questions
What Are Molecular Motors?
Molecular motors are proteins that convert chemical energy into mechanical force, enabling movement and work within biological systems. They are essential for various cellular processes, including muscle contraction, cell division, and the transport of molecules across cells.
How Do Linear and Rotary Molecular Motors Differ?
The main difference between linear and rotary molecular motors is their motion. Linear motors move in a straight line, utilizing a track-like structure within the cell, whereas rotary motors rotate around an axis, using this rotation to perform work, such as synthesizing ATP or propelling bacterial movement.
Why Are Molecular Motors Important?
Molecular motors are crucial for the survival and function of living organisms. They are involved in key biological processes, such as transporting nutrients and organelles within cells, facilitating cell movement, and generating force for muscle contractions. Understanding molecular motors helps scientists develop new treatments for diseases and create advanced technologies.
Can Molecular Motors Be Used in Technology?
Yes, molecular motors have promising applications in nanotechnology and medicine. They can be harnessed to build nanoscale devices for targeted drug delivery, act as components in nanomachines, and inspire the design of new materials with dynamic, self-assembling properties.
Conclusion
Molecular motors, in their linear and rotary forms, exemplify nature’s ingenuity in designing mechanisms that are both efficient and versatile. The understanding of these tiny engines not only sheds light on fundamental biological processes but also opens avenues for technological innovations that were once the realm of science fiction. By mimicking or leveraging these natural processes, researchers can develop novel applications in medicine, robotics, and materials science.
The journey of exploring and harnessing the potential of molecular motors is an exciting frontier in both biology and engineering. As our knowledge deepens and technologies advance, the possibilities for application seem almost limitless, promising a future where molecular motors play a central role in solving some of humanity’s most complex challenges.