Molecular probes are essential tools in scientific research, offering unique insights into the complexities of biological and chemical processes. These probes, which can be either radioactive or nonradioactive, play a pivotal role in a variety of applications, from medical diagnostics to environmental monitoring. The choice between these two types often depends on specific research requirements, including sensitivity, safety, and resolution.
Radioactive probes consist of a biomolecule labeled with a radioactive isotope, whereas nonradioactive probes are tagged with a stable, non-emitting label. While radioactive probes are highly sensitive and can detect minute biological changes, nonradioactive probes offer safer handling and longer shelf-life, making them suitable for different types of research environments.
The distinction between radioactive and nonradioactive probes lies in their make-up, usage, and impact on both health and the environment. Researchers choose between these based on factors such as required sensitivity, the nature of the experiment, and regulatory guidelines. This article explores these differences in depth, focusing on how they influence the scientific outcomes in various research scenarios.
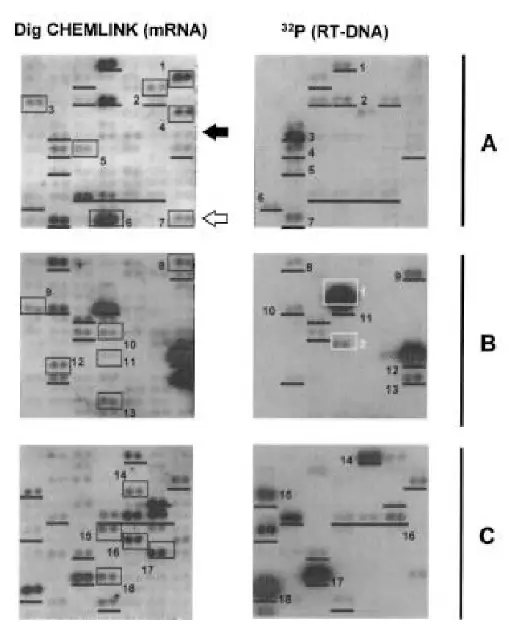
Basic Concepts
Probes Defined
Definition and Purpose
Molecular probes are specialized tools used in scientific research to detect, track, or quantify specific biological, chemical, or physical processes. They are designed to bind selectively to their target, allowing researchers to gain detailed insights into cellular function, genetic expression, and more.
Types of Probes in Research
Probes vary widely in type and function. Key categories include:
- Fluorescent probes which emit light upon excitation
- Chromogenic probes which change color in the presence of a target
- Radioactive probes that use isotopes to trace processes
- Enzymatic probes that produce a signal through enzymatic activity
Radioactivity Explained
What is Radioactivity?
Radioactivity involves the emission of ionizing radiation as unstable atoms decay. This process is a cornerstone in many scientific applications due to its sensitivity and precision.
Uses in Probes
In the context of probes, radioactivity is utilized because of its ability to provide extremely sensitive and quantifiable results in complex biological systems. It is especially valuable in:
- Tracking molecular interactions
- Diagnosing diseases
- Monitoring therapeutic responses
Radioactive Probes
Core Features
Composition and Structure
Radioactive probes consist of a molecule such as DNA, RNA, or a protein labeled with a radioactive isotope, commonly phosphorus-32 or sulfur-35. These isotopes are incorporated into the probe molecule, allowing it to be tracked via radiation.
Mechanism of Action
The mechanism is straightforward: the probe binds to its target, and the radioactive decay is measured. This measurement tells researchers the location and quantity of the target molecule within the sample.
Applications
Use in Medical Diagnostics
Radioactive probes are pivotal in medical diagnostics, particularly in:
- Detecting cancerous cells using specific biomarkers
- Assessing organ function such as thyroid activity
Role in Genetic Engineering
In genetic engineering, these probes are crucial for:
- Gene localization identifying where specific genes are located on chromosomes
- Mutation detection finding alterations in genetic sequences
Safety Concerns
Health Risks
Handling radioactive materials involves risks such as:
- Radiation exposure which can cause cellular damage and long-term health issues
- Contamination which can lead to spread of radioactive materials
Handling and Regulations
Strict protocols govern the use of radioactive probes:
- Safety training for all personnel
- Usage of protective equipment such as lead shields and gloves
- Regulatory compliance with both disposal and usage guidelines
Nonradioactive Probes
Core Features
Composition and Nonradioactive Elements
Nonradioactive probes may use fluorescent tags or biotin molecules attached to DNA, RNA, or proteins. These tags emit signals detected by specialized instruments without the risks associated with radioactivity.
Mechanism of Action
The action of nonradioactive probes involves the emission of light or other detectable signals when the probe binds to its target, providing a safe and effective means of detection that does not decay over time.
Applications
Biotechnology Uses
In biotechnology, nonradioactive probes help in:
- Cloning by identifying gene sequences
- Protein analysis through Western blotting or ELISA
Environmental Monitoring
These probes are used to monitor pollutants in ecosystems, detecting toxic substances at molecular levels.
Advantages
Safety and Handling
Nonradioactive probes offer significant safety benefits:
- No special storage requirements
- Reduced health risks making them preferable in educational settings
Stability and Storage
These probes are stable over long periods, which reduces costs related to:
- Reordering
- Waste management due to longer shelf lives
Comparative Analysis
Performance Comparison
Sensitivity and Specificity
When comparing radioactive and nonradioactive probes, sensitivity and specificity are crucial metrics. Radioactive probes are highly sensitive, capable of detecting minute amounts of a target molecule, a feature particularly valuable in low-abundance scenarios. Their specificity, however, can sometimes be compromised by the background noise created by nonspecific binding or decay.
Nonradioactive probes, while generally less sensitive than their radioactive counterparts, offer enhanced specificity with lower background signals. This makes them particularly effective in applications requiring precise targeting without interference, such as in detailed cellular or tissue imaging.
Duration and Decay
Radioactive probes are limited by the half-life of the isotope used, which can range from hours to weeks, affecting the duration of the experiment. Their signal weakens over time, necessitating precise timing in experimental design.
In contrast, nonradioactive probes do not suffer from decay over time, maintaining stable signal intensity and allowing for extended study periods. This stability is advantageous for longitudinal studies where consistent monitoring over time is required.
Cost Implications
Initial Costs
The initial costs of radioactive probes are often higher due to the need for isotopes, which can be expensive and require special handling and storage facilities. Additionally, the equipment needed to detect radiation is typically more sophisticated and costly.
Nonradioactive probes, while potentially less expensive upfront, may involve significant investment in specialized detection equipment, such as fluorescence microscopes or chemiluminescence detectors, depending on the type of label used.
Long-term Expenditures
The long-term costs associated with radioactive probes include safety measures, disposal of radioactive waste, and recurring purchases of isotopes due to their decay. These factors can significantly increase the total cost of ownership.
Nonradioactive probes tend to have lower long-term costs, primarily because they do not require expensive waste management protocols and have longer shelf lives, reducing the frequency of re-purchase.
Environmental Impact
Waste Management
Radioactive waste management is a major environmental concern. The disposal of radioactive probes must comply with strict regulatory guidelines to prevent environmental contamination, requiring substantial resources and planning.
Nonradioactive probes produce less hazardous waste, making their disposal simpler and less costly. This aspect significantly reduces their environmental footprint compared to radioactive probes.
Regulatory Compliance
Compliance with regulations for radioactive probes is both rigorous and costly, involving multiple government agencies and often necessitating extensive documentation and periodic audits.
Nonradioactive probes benefit from generally simpler compliance requirements, which can reduce bureaucratic burdens and facilitate quicker and less expensive project approvals.
Future Directions
Technological Advances
Innovations in Probe Design
The future of probe technology lies in the development of new materials and labeling techniques that combine the high sensitivity of radioactive methods with the safety and stability of nonradioactive approaches. Innovations such as quantum dots and advanced fluorescent compounds are already making an impact.
Impact on Research Efficiency
These technological advancements promise to dramatically increase the efficiency of research by reducing the time needed for experiments and enhancing the quality of data obtained, potentially revolutionizing fields such as molecular biology, genetics, and biochemistry.
Shifts in Usage
Trends Towards Nonradioactivity
There is a noticeable shift in the scientific community towards nonradioactive probes, driven by improvements in nonradioactive technologies and increasing regulatory and safety concerns associated with radioactive materials. This trend is expected to continue as the benefits of nonradioactive approaches become more pronounced.
Predicted Changes in Regulations
As nonradioactive technologies become more prevalent, regulatory bodies are likely to adjust standards to promote safer practices, possibly tightening regulations on radioactive materials while streamlining approvals for nonradioactive methods. These changes will further encourage the adoption of nonradioactive probes in scientific research.
Frequently Asked Questions
What are molecular probes?
Molecular probes are specialized molecules designed to bind to specific targets within a biological or chemical system, allowing researchers to track or quantify various processes or components. These probes can be radioactive, containing isotopes that emit radiation, or nonradioactive, tagged with fluorescent or other detectable markers.
How do radioactive probes work?
Radioactive probes work by incorporating radioisotopes that emit radiation detectable by specific imaging equipment. This radiation signals the presence and distribution of the target molecule in a sample, useful in fields like oncology and genetics for tracing and diagnosing conditions.
Are nonradioactive probes safer?
Nonradioactive probes are generally considered safer than radioactive ones because they do not involve radiation exposure. This reduces health risks for laboratory personnel and eliminates the need for special disposal procedures associated with radioactive waste.
Why choose nonradioactive over radioactive probes?
Nonradioactive probes are often chosen over radioactive ones due to their safety, ease of handling, and stability. They do not degrade over time as radioactive isotopes do, and they pose no risk of radioactive contamination, making them ideal for prolonged and repetitive experiments.
Can radioactive probes be used in clinical settings?
Radioactive probes are widely used in clinical settings, especially in diagnostic imaging like PET scans. These probes help in accurately diagnosing diseases by highlighting areas of metabolic or chemical activity in the body, although their use is strictly regulated to minimize exposure risks.
Conclusion
The decision between using radioactive or nonradioactive probes hinges on specific research needs, balancing factors like sensitivity, safety, and environmental impact. Each type of probe has its advantages and limitations, influencing their suitability for various applications. As technology advances, the development of new probe types that combine the best features of both radioactive and nonradioactive probes could revolutionize the field, offering more precise and safer options for researchers and clinicians alike.
In conclusion, understanding the differences between these probes not only enhances scientific inquiry but also guides researchers in selecting the optimal tools for their specific needs. This knowledge ensures the continued advancement of scientific research, pushing the boundaries of what is possible in diagnostics, treatment, and environmental monitoring.