Meiosis is a fundamental biological process, crucial for the production of gametes in sexually reproducing organisms. This cellular division not only halves the genetic material but also introduces genetic diversity through phases such as zygotene and pachytene. These stages are key in the complex choreography of chromosome pairing and genetic recombination.
The zygotene and pachytene stages of meiosis differ primarily in their cellular activities and roles. Zygotene marks the early phase where chromosomes begin pairing together through a process called synapsis. In contrast, pachytene is the phase where the synapsed chromosomes are tightly aligned, allowing for the exchange of genetic material, a process vital for genetic diversity.
During meiosis, the accurate execution of events in both zygotene and pachytene is critical for the correct segregation of chromosomes. Errors in these stages can lead to genetic disorders and have profound implications on fertility and developmental biology. Understanding these stages helps scientists and medical professionals manage and investigate a range of genetic conditions.
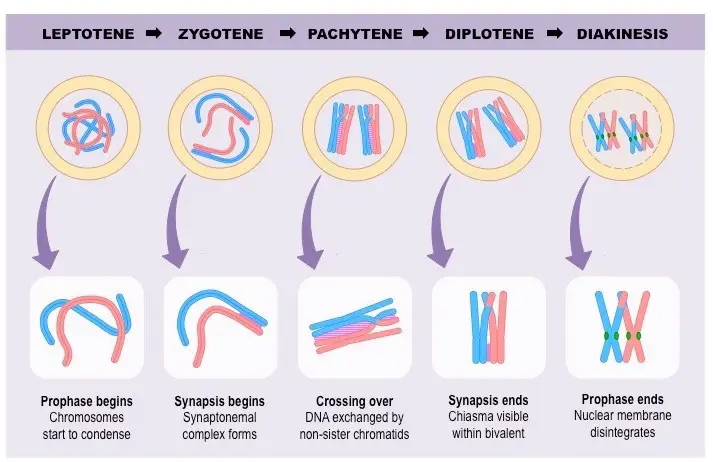
Meiosis Basics
Definition and Significance
Meiosis is a special type of cell division that reduces the chromosome number by half, creating four haploid cells from one diploid cell. Each of these cells contains unique genetic information, which is crucial for sexual reproduction. This process ensures that when sperm and egg unite, the resulting offspring has a complete set of chromosomes. Meiosis is fundamental in maintaining genetic diversity, which is key to the survival and evolution of species.
Stages Overview
Meiosis consists of two sequential rounds of cell division, meiosis I and meiosis II, but only one round of DNA replication. It is divided into several phases:
- Prophase I: Chromosomes condense, and homologous chromosomes pair up.
- Metaphase I: Paired chromosomes align at the cell’s equator.
- Anaphase I: Homologous chromosomes separate to opposite poles.
- Telophase I and Cytokinesis: The cell divides into two.
- Prophase II: No further chromosome replication occurs.
- Metaphase II, Anaphase II, and Telophase II: Similar to mitosis, chromosomes align, separate, and the cells divide, resulting in four haploid daughter cells.
Zygotene Phase
Definition of Zygotene
Zygotene is the second stage of prophase I in meiosis, following leptotene. During zygotene, chromosomes start to pair up, a process essential for the next steps of meiosis.
Key Processes
- Synapsis: Homologous chromosomes begin to pair closely along their lengths.
- Formation of the Synaptonemal Complex: A protein structure forms between homologous chromosomes, stabilizing their pairing.
Biological Role
The biological role of the zygotene phase is to prepare chromosomes for recombination. By aligning homologous chromosomes, zygotene sets the stage for the exchange of genetic material, which is crucial for genetic diversity.
Pachytene Phase
Definition of Pachytene
Pachytene is the third stage of prophase I in meiosis. It follows zygotene and is marked by the full establishment of the synaptonemal complex.
Key Features
- Complete Synapsis: Homologous chromosomes are fully paired.
- Crossing Over: Genetic material is exchanged between chromosome pairs.
- Visible Chiasmata: Sites of crossing over are visible under a microscope.
Biological Implications
Pachytene is critical for genetic recombination, which enhances genetic diversity. It ensures that the genetic information passed on to the offspring includes a mix from both parents, contributing to the uniqueness of each individual.
Comparing Zygotene and Pachytene
Similarities Overview
Zygotene and pachytene are both stages of prophase I in meiosis where homologous chromosomes interact. They are crucial for the process of genetic recombination.
Distinctive Characteristics
- Timing: Zygotene precedes pachytene and involves the initiation of synapsis, whereas pachytene completes it.
- Processes: Zygotene is about beginning the pairing and synapsis, while pachytene involves the full establishment of the synaptonemal complex and the actual exchange of genetic material.
Diagrammatic Representation
A diagram here would illustrate the progressive changes in chromosome structure and interaction from zygotene to pachytene, showing synapsis and the formation of chiasmata visually.
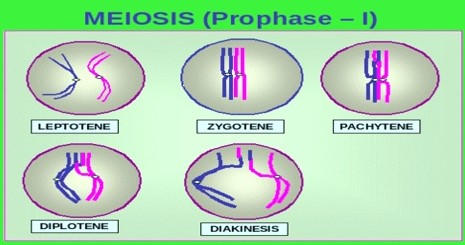
Genetic Implications
Impact on Genetic Variation
Genetic variation is the cornerstone of evolutionary biology and is critical for species survival. The stages of zygotene and pachytene in meiosis are pivotal in fostering this variation. During zygotene, homologous chromosomes begin to pair up, setting the stage for recombination, which is executed in pachytene. This recombination during pachytene allows for the exchange of genetic segments between chromosomes. Such exchanges introduce new gene combinations into the gametes, significantly increasing the genetic diversity within a population.
Importance in Genetic Disorders
The processes that occur during zygotene and pachytene also have profound implications for genetic health. Errors during these phases can lead to misalignments or improper genetic recombination, resulting in mutations or chromosomal abnormalities. Such errors are linked to various genetic disorders, including Down syndrome, Klinefelter syndrome, and Turner syndrome. These conditions arise from non-disjunction or other chromosomal mishaps during meiosis, highlighting the critical role of precise chromosomal pairing and separation.
Research and Discoveries
Historical Perspectives
The discovery of meiosis dates back to the late 19th century, with scientists like Oscar Hertwig and Edouard Van Beneden observing cell division in animal cells, which later contributed to identifying meiotic processes. The introduction of the synaptonemal complex and the understanding of genetic recombination were significant milestones in cytogenetics, initially observed through light microscopy and later through more advanced imaging techniques.
Recent Studies
In recent years, molecular biology and genetic engineering have shed new light on the mechanisms of meiosis. Advanced techniques such as CRISPR and fluorescence in situ hybridization (FISH) have allowed scientists to study the specific roles and behaviors of genes during zygotene and pachytene. Recent studies focus on understanding the molecular triggers that initiate synapsis and the enzymatic activities involved in crossing over, providing deeper insights into how genetic diversity is achieved and maintained.
Practical Applications
Medical Relevance
The understanding of meiosis, particularly zygotene and pachytene phases, has crucial medical applications. It aids in the development of treatments for infertility and genetic disorders. For instance, knowing how chromosomes misalign can help in devising diagnostic tools and therapeutic strategies to manage or correct these anomalies before they impact fertility or lead to congenital disabilities.
Agricultural Impact
Agriculturally, the knowledge of genetic recombination during meiosis is used to enhance crop breeding strategies. By manipulating the conditions under which crossing over occurs during pachytene, agronomists can create plant varieties with desirable traits such as drought resistance, improved yield, and disease resistance. This genetic manipulation not only ensures food security but also promotes sustainable agricultural practices by reducing the need for chemical inputs like pesticides and fertilizers.
Frequently Asked Questions
What is Meiosis?
Meiosis is a type of cell division that reduces the chromosome number by half, creating four haploid cells, each genetically distinct from the parent cell. This process is essential for sexual reproduction and contributes to genetic diversity.
What happens during Zygotene?
During zygotene, chromosomes begin to pair up with their corresponding homologous chromosomes. This pairing is facilitated by the formation of a protein structure called the synaptonemal complex, which helps align the chromosomes precisely.
What is the significance of Pachytene?
Pachytene is significant as it is the stage where crossing over occurs between homologous chromosomes. This genetic exchange is crucial for recombination, which increases genetic diversity in the resulting gametes.
How do Zygotene and Pachytene contribute to genetic diversity?
Zygotene and pachytene contribute to genetic diversity by ensuring chromosomes pair and exchange genetic material accurately. This recombination and the subsequent separation of homologous chromosomes increase the genetic variability among offspring.
Conclusion
The exploration of zygotene and pachytene reveals their essential roles in the life cycle of sexually reproducing organisms. By understanding these stages, researchers can better grasp how genetic diversity is achieved and maintained. Additionally, insights gained from studying these meiotic stages contribute to advancements in genetic therapies and fertility treatments.
As science continues to uncover more about these critical phases of meiosis, the potential for new discoveries in genetic medicine and agriculture remains vast. The ongoing research and interest in zygotene and pachytene not only enhance our foundational biological knowledge but also improve practical applications in various fields.