Fluxionality and tautomerism are two fascinating concepts in the realm of chemistry, each playing a pivotal role in how scientists understand and manipulate molecular structures. These processes involve dynamic changes at the molecular level but differ fundamentally in their mechanisms and implications. By examining these differences, chemists can gain deeper insights into molecular behavior and stability.
Fluxionality refers to the ability of certain molecular structures to undergo dynamic changes that allow atoms or groups of atoms within a molecule to interchange positions without breaking chemical bonds. Tautomerism, on the other hand, involves the shift of a proton within a molecule and a corresponding change in the position of a double bond, resulting in different isomeric forms. Both processes are crucial for understanding chemical reactivity and the physical properties of substances.
While fluxionality often impacts the entire molecule’s conformation and is typically observed in metal-organic frameworks and some organic molecules, tautomerism is a critical behavior in organic chemistry and biochemistry, influencing the stability and biological activity of molecules. The study of these processes not only enriches fundamental chemistry but also aids in the development of new pharmaceuticals, materials, and catalysts.
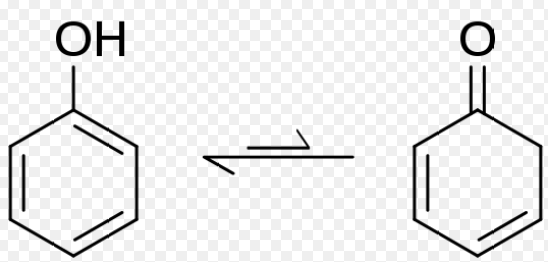
Fluxionality Explained
What is Fluxionality?
Fluxionality refers to the dynamic and reversible process where atoms within a molecule rearrange themselves around their bonding framework. This fascinating aspect of chemistry highlights the fluid nature of molecular structures, particularly in coordination and organometallic chemistry. In essence, fluxional molecules exhibit movements that allow them to adapt to different geometrical configurations without breaking chemical bonds.
Definition and Basics
At its core, fluxionality involves the continuous interchange of positional or orientation bonds within a molecule, facilitated by the movement of atoms or groups of atoms. This process is generally observed in molecules containing metal centers, where ligands around the metal atom shift positions through a variety of mechanisms.
Examples of Fluxionality
Common Examples in Chemistry
- Boranes: Diborane (B2H6) showcases fluxionality through the rapid reorganization of its hydrogen atoms.
- Ferrocene: In this organometallic compound, the cyclopentadienyl rings rotate freely about the iron center.
- Transition Metal Carbonyls: Molecules like Fe(CO)5 demonstrate the movement of carbonyl groups around the metal center.
Mechanism Behind Fluxionality
How Fluxionality Occurs at the Molecular Level
The mechanism of fluxionality can be explained through several pathways, depending on the molecule in question. Typically, these movements are facilitated by factors such as:
- Thermal energy providing sufficient force for atoms to overcome energy barriers between different configurations.
- Symmetry and bonding characteristics that allow for smooth transitions between configurations.
Tautomerism Overview
Definition of Tautomerism
Tautomerism is a chemical phenomenon where a compound exists in two (or more) readily interconvertible structures differing notably in the position of protons and electrons. The two forms, known as tautomers, are in dynamic equilibrium and involve the shift of chemical bonds and atoms, typically hydrogen and a double bond.
Basic Understanding
The concept of tautomerism is particularly significant in organic chemistry, where it influences the physical and chemical properties of compounds, including their stability, reactivity, and biological activity. This dynamic equilibrium is critical in many biochemical processes and drug design.
Tautomerism Types
Keto-enol
The most familiar type of tautomerism involves the keto and enol forms, where a carbonyl group (C=O) and an adjacent alcohol group (C-OH) interconvert.
Amine-imine
Another common form involves nitrogen compounds, where an amine (NH2) converts to an imine (NH) group.
Others
- Lactam-lactim: Involves cyclic compounds where a lactam (a cyclic amide) converts to a lactim (a cyclic imine).
- Ring-chain: Some sugars exhibit this form, where open-chain forms of sugars convert to cyclic forms.
Mechanism of Tautomerism
Step-by-step Process
- Proton Shift: The proton in the molecule relocates from one atom to another, usually from oxygen to carbon in keto-enol tautomerism.
- Double Bond Reformation: As the proton shifts, the double bond’s position changes, re-establishing the stability of the molecule.
- Equilibrium Establishment: The forward and reverse reactions occur at equal rates, establishing a dynamic equilibrium between the tautomeric forms.
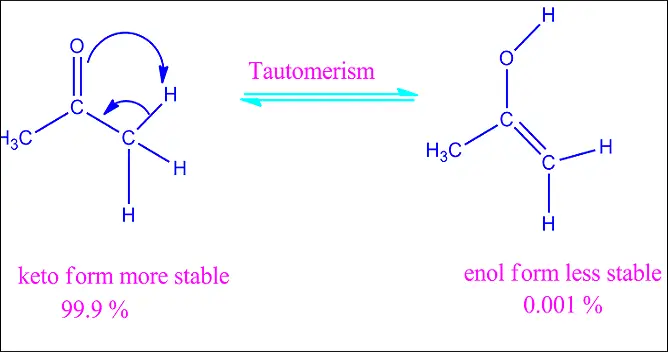
Key Differences
Structural Changes
Comparison of Structural Dynamics
Fluxionality and tautomerism both involve changes in molecular structure, but the nature and scope of these changes differ significantly. In fluxionality, the structural changes are generally confined to the rearrangement of ligands around a central metal atom without breaking any chemical bonds. For example, in metal carbonyl clusters, ligands like CO groups move in a controlled manner that allows the molecule to explore different configurations while remaining intact.
In contrast, tautomerism involves the actual shift of a proton and a corresponding rearrangement of double bonds, which fundamentally changes the molecule’s chemical structure. This transformation leads to the creation of distinctly different forms, known as tautomers, which can have different chemical properties and reactivities.
Energy States
Energy Requirements and Stability
The energy landscapes for fluxionality and tautomerism are also distinct. Fluxional behavior typically occurs with relatively low energy barriers, allowing the molecule to fluctuate between configurations at room temperature or with mild heating. This characteristic enables the molecule to adapt its shape easily to different environments or catalytic processes.
Tautomerism, however, often involves higher energy barriers, depending on the strength of the bonds being broken and formed. The stability of tautomers can be markedly different, and the position of the equilibrium between tautomers can be influenced by factors such as solvent, temperature, and pH.
Occurrence and Conditions
Environmental Factors Affecting Each
The environmental conditions that favor fluxionality versus tautomerism vary. Fluxional processes are often favored in coordination complexes with flexible ligand environments and in situations where rapid ligand exchange can facilitate catalysis or transport processes. Tautomerism is more commonly observed in organic compounds under conditions where hydrogen bonding and proton solvation can occur, such as in aqueous solutions or biological systems.
Applications and Implications
In Organic Synthesis
How They Influence Synthesis
Fluxionality and tautomerism significantly influence organic synthesis strategies. The ability of fluxional molecules to adapt their structures can be exploited in catalyst design, where the flexibility of the catalyst enhances its effectiveness and selectivity. Tautomerism plays a critical role in the synthesis of pharmaceuticals and biologically active molecules, where different tautomeric forms can have different biological activities and metabolic stabilities.
In Biological Systems
Role in Biochemistry and Pharmaceuticals
In biological systems, tautomerism is crucial for the function of many biomolecules. Nucleic acids, for example, exhibit tautomerism, which can affect the base pairing in DNA and result in mutations. This has profound implications for drug design, as different tautomeric forms of a drug molecule may interact differently with biological targets.
Technological Applications
Usage in Material Science
Both fluxionality and tautomerism find applications in material science. Materials designed with fluxional properties can exhibit reversible changes in their structure, leading to applications in smart materials and sensors. Tautomerism can be used to design photochromic materials, where changes in light conditions trigger changes in the material’s color or optical properties.
Challenges and Considerations
Measurement and Observation
Techniques and Tools for Studying
Studying fluxionality and tautomerism presents unique challenges. Techniques such as nuclear magnetic resonance (NMR) spectroscopy, infrared spectroscopy, and X-ray crystallography are crucial for observing these phenomena. Advanced computational models also play a key role in predicting and understanding the behavior of fluxional and tautomeric systems.
Predictive Challenges
Computational and Theoretical Approaches
Predicting when and how fluxionality or tautomerism will occur in a molecule involves complex theoretical calculations. Quantum chemical methods are often used to map out the potential energy surfaces of molecules to understand the barriers to fluxional or tautomeric change. These computational studies are essential for designing molecules with desired properties and for understanding fundamental aspects of chemical reactivity.
Frequently Asked Questions
What is Fluxionality?
Fluxionality is a phenomenon observed in certain molecules where parts of the molecule rearrange themselves around a single bonding framework. This behavior is crucial for understanding the flexibility and dynamic nature of molecular structures in complex chemical systems.
How Does Tautomerism Affect Chemical Properties?
Tautomerism can significantly affect the chemical properties of a substance, such as its acidity, basicity, and reactivity. This is because the shift in hydrogen atoms and double bonds can create different functional groups within the same molecule.
Are Fluxional Molecules Common in Nature?
Fluxional molecules are less common in nature than in synthetic environments but play vital roles in some biological and environmental systems. Their ability to adapt to different shapes makes them essential in certain biological mechanisms.
What are the Technological Applications of Tautomerism?
Tautomerism finds applications in drug design and development, where the tautomeric form of a molecule can influence its therapeutic effectiveness and interaction with biological targets.
Conclusion
In conclusion, fluxionality and tautomerism represent key aspects of molecular dynamics that have profound implications across chemistry and related disciplines. Understanding these processes enhances our ability to design more effective drugs, develop new materials, and solve complex biological problems. Through ongoing research and technological advances, the exploration of these dynamic changes continues to unveil new possibilities in science.
The study of fluxionality and tautomerism not only challenges our foundational understanding of chemical stability but also opens up pathways for innovative applications in various fields. As researchers delve deeper into the mechanics of these molecular transformations, the potential for new discoveries remains vast and ever-expanding.