The relationship between inductance and voltage is a cornerstone of electromagnetic theory, shaping the way we understand and utilize electricity. This interplay is critical in designing and operating a wide array of electrical systems, from simple circuits to complex power grids. It reflects fundamental principles of physics that dictate how electrical energy is stored and transformed within magnetic fields created by electric currents.
Inductance, the property of an electrical conductor by which a change in current through it induces an electromotive force (voltage) in both the conductor itself (self-inductance) and in any nearby conductors (mutual inductance), is intrinsically linked to voltage. The induced voltage is proportional to the rate of change of current over time. This relationship is encapsulated in Faraday’s law of electromagnetic induction, which serves as a fundamental equation in electromagnetism.
Understanding the dynamics of inductance and voltage unveils the principles behind the functioning of inductors in circuits, how they store energy, filter signals, and affect the flow of electrical current. These insights are pivotal for the development of efficient energy storage solutions, effective signal processing techniques, and the reliable transmission of power across vast networks. By exploring the nuances of this relationship, we uncover the mechanisms that underpin modern electrical engineering and technology.
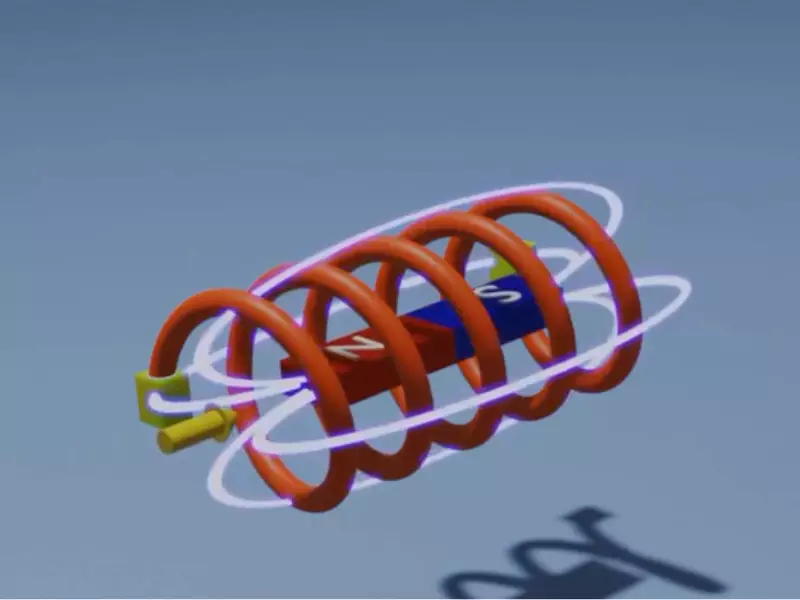
Basics of Inductance
Definition and Fundamentals
Inductance is a fundamental property of electrical circuits that describes how a changing electric current induces an electromotive force, or voltage, in the conductor and potentially in nearby conductors. This property is pivotal in the operation of many electrical devices, affecting how energy is stored and transferred within circuits.
How Inductance Works
At its core, inductance is about energy storage in a magnetic field. When electric current flows through a conductor, it generates a magnetic field around it. If the current changes—either in magnitude or direction—the magnetic field changes accordingly. This changing magnetic field, in turn, induces a voltage in the conductor. This phenomenon is known as electromagnetic induction and is governed by Faraday’s law.
Types of Inductors
Inductors, the components designed to exhibit inductance, come in various types to suit different applications:
- Air-core inductors: Use air as the core material, offering lower inductance values but minimal losses.
- Ferrite-core inductors: Incorporate ferrite to achieve higher inductance levels, useful in high-frequency applications.
- Toroidal inductors: Characterized by their donut shape, these inductors offer high efficiency and reduced electromagnetic interference.
Basics of Voltage
Voltage Explained
Voltage, also known as electric potential difference, is the force that drives electric current through a conductor. It’s a measure of the potential energy difference per unit charge between two points in an electrical field. Simply put, voltage is what makes electric charges move, analogous to pressure in a water pipe.
Role in Electrical Circuits
In electrical circuits, voltage is the driving force that enables current to flow, powering devices and systems. It’s what pushes electrons through conductors, resistors, and other circuit components, enabling the conversion of electrical energy into other forms of energy, such as light, heat, or mechanical power.
Types of Voltage
Voltage can be classified into two main types:
- Direct Current (DC) voltage: Remains constant over time, typically used in batteries and power supplies.
- Alternating Current (AC) voltage: Changes magnitude and direction periodically, characteristic of power grids and home outlets.
Inductance and Voltage Relationship
Theoretical Background
The relationship between inductance and voltage is governed by Lenz’s law, which states that the induced voltage will be in such a direction as to oppose the change in current that created it. This fundamental principle underlines the role of inductance in resisting changes in current flow, thereby playing a crucial role in circuit behavior.
Formula and Calculation
The mathematical relationship between inductance (L), the rate of change of current (����dtdI), and the induced voltage (V) is given by:
�=�×����V=L×dtdI
This equation highlights how a faster change in current or a higher inductance value results in a greater induced voltage.
Practical Examples
- In transformers, varying the current in the primary coil induces a voltage in the secondary coil, transforming voltage levels.
- Chokes and filters use inductance to block or reduce high-frequency AC signals, allowing DC or low-frequency signals to pass.
Factors Influencing Inductance and Voltage
Core Material
The material of the inductor’s core significantly impacts its inductance. Materials with higher magnetic permeability, such as iron or ferrite, enhance the magnetic field, thus increasing inductance.
Coil Turns
The number of turns in the coil also affects inductance. More turns increase the magnetic field for a given current, thereby increasing inductance.
Frequency of Current
The frequency of the alternating current influences both inductance and the resultant voltage. Higher frequencies cause a greater rate of change in the magnetic field, which can increase the induced voltage and affect the inductor’s behavior, such as in the case of skin effect and proximity effect.
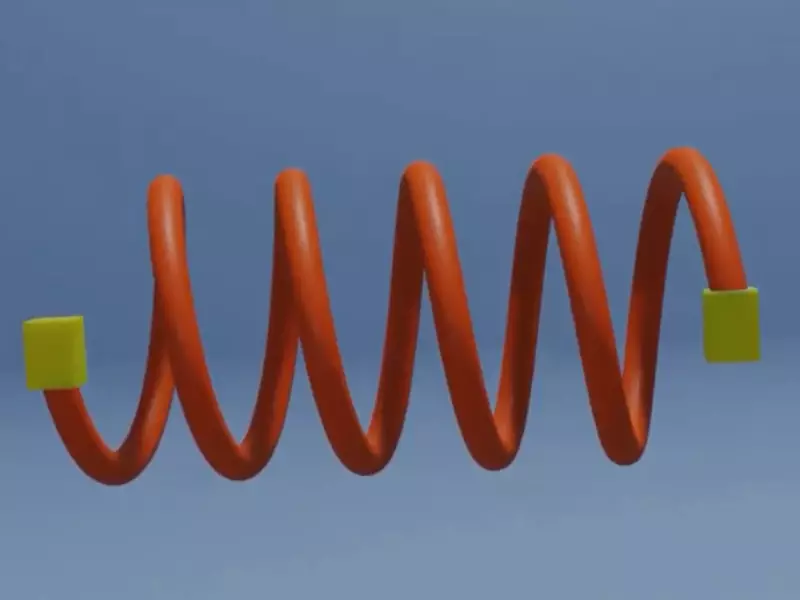
Measurement Techniques
Accurate measurement is fundamental in electrical engineering, ensuring devices and systems function as intended. This section explores tools and methods for measuring inductance and voltage, highlighting the importance of precision.
Tools for Measuring Inductance
Inductance measurement requires specialized tools to evaluate the effectiveness of inductors in circuits. Key instruments include:
- LCR Meters: These devices measure inductance (L), capacitance (C), and resistance (R), providing comprehensive data about the component under test.
- Impedance Analyzers: Used for high-precision measurements, these analyzers can detail inductor performance over a range of frequencies.
- Oscilloscopes with Inductance Measurement Capabilities: Advanced oscilloscopes can measure inductance by analyzing changes in voltage and current over time.
Using these tools, engineers can determine inductance values accurately, ensuring components meet design specifications.
Voltage Measurement Methods
Measuring voltage is critical for diagnosing and troubleshooting electrical systems. Common methods include:
- Multimeters: Versatile tools that measure voltage, current, and resistance. They are essential for any electrical engineering work.
- Voltmeters: Specifically designed for measuring voltage, providing more precision for this specific task.
- Oscilloscopes: Beyond inductance measurement, oscilloscopes can visualize voltage waveforms over time, offering insights into the behavior of electrical signals.
Importance of Precision in Measurements
Precision in measurements guarantees that electrical components and systems operate safely and efficiently. It aids in:
- Avoiding Component Failure: Accurate measurements help identify components not meeting specifications, preventing potential failures.
- Enhancing Efficiency: Precision ensures systems are optimized for performance, reducing energy waste.
- Ensuring Safety: Accurate voltage measurements prevent overvoltage conditions, protecting both the device and the user.
Applications in Technology
Inductance and voltage play vital roles across various technology sectors. Their applications in energy storage, signal processing, and power transmission are critical for modern innovations.
Energy Storage
Inductors are key in energy storage technologies, particularly in converting and managing power supplies. Applications include:
- Switched-mode Power Supplies: These devices use inductors to store energy temporarily for efficient power conversion.
- Electric Vehicles: Inductors in EVs manage the flow of electricity between batteries and motors, crucial for performance and efficiency.
Signal Processing
In signal processing, inductors filter unwanted frequencies, maintaining signal integrity. They are used in:
- Radio Frequency (RF) Filters: Inductors block specific frequencies, allowing clear communication signals.
- Audio Equipment: High-quality audio systems use inductors to eliminate noise, improving sound clarity.
Power Transmission
Inductance affects power transmission in significant ways, especially in:
- Transformers: Key components in electrical grids, transformers use inductance to transfer energy efficiently across circuits at different voltages.
- Wireless Charging: Inductive coupling transfers power wirelessly, a technology used in smartphones and electric toothbrushes.
Challenges and Solutions
While inductance and voltage are fundamental to electrical engineering, they introduce challenges that require innovative solutions.
Losses and Efficiency
Energy losses, primarily from resistance in inductors, reduce system efficiency. Solutions include:
- Using High-permeability Core Materials: Reduces the core losses in inductors and transformers.
- Optimizing Coil Design: Minimizes resistance and enhances the inductance-to-resistance (L/R) ratio, improving efficiency.
Mitigation Techniques
To combat inefficiencies and losses, engineers employ various mitigation techniques, such as:
- Active Power Factor Correction: Improves the efficiency of power conversion systems, reducing losses.
- Thermal Management: Ensures that components operate within safe temperature ranges, preventing overheating and energy waste.
Recent Advances
Technological advancements continue to address the challenges associated with inductance and voltage. Innovations include:
- Nanocrystalline Core Materials: Offer higher permeability and lower losses, enhancing efficiency in transformers and inductors.
- Smart Grid Technologies: Use advanced metering and control to improve the efficiency and reliability of power transmission and distribution.
Frequently Asked Questions
How does inductance affect a circuit?
Inductance affects a circuit by opposing changes in the current flow, storing energy in a magnetic field when the current is increasing and releasing energy back into the circuit when the current decreases. This characteristic is crucial for applications like filters, where inductors block high-frequency signals, or in energy storage, where they maintain a steady flow of electricity.
What determines the voltage induced by inductance?
The voltage induced by inductance is determined by the rate of change of current through the conductor and the inductance value of the conductor itself. The faster the current changes, or the higher the inductance, the greater the induced voltage. This principle is described mathematically by Faraday’s law of electromagnetic induction.
Can inductance be changed in a circuit?
Yes, inductance in a circuit can be changed by altering the physical properties of the inductor. This includes changing the core material to one with a different magnetic permeability, modifying the number of coils or turns in the inductor, or adjusting the shape or size of the coil. Each of these factors directly influences the inductance value, allowing for precise control over the circuit’s behavior.
Conclusion
The intricate relationship between inductance and voltage underpins much of what is possible in the realm of electrical engineering and technology. Through understanding how these two principles interact, we are better equipped to design and implement more efficient and effective electrical systems. This knowledge not only advances our technical capabilities but also deepens our appreciation for the fundamental laws of physics that govern our world.
As we look to the future, the ongoing exploration of inductance and voltage, along with advancements in material science and electronic engineering, promises to usher in new innovations and improvements in energy storage, signal processing, and power transmission. The principles that once sparked the electrical revolution continue to illuminate the path forward, demonstrating the timeless nature of these fundamental concepts.