Coordination complexes stand at the crossroads of inorganic chemistry, influencing both the fundamental understanding of chemical bonding and the development of practical applications ranging from catalysis to medicine. These complexes feature a central metal ion surrounded by molecules or anions, known as ligands, in a specific geometric arrangement. The structure of these complexes plays a crucial role in determining their chemical behavior and physical properties.
The difference between square planar and tetrahedral complexes lies primarily in their geometric configuration. Square planar complexes are characterized by a central metal ion surrounded by ligands at the corners of a square on the same plane, while tetrahedral complexes have ligands located at the corners of a tetrahedron. These configurations result from the metal’s electronic structure, the type of ligands involved, and the overall coordination number, which significantly impacts the complex’s reactivity, stability, and color.
Understanding these differences is vital for chemists and researchers as it aids in predicting the properties of unknown compounds, designing new materials with desired characteristics, and advancing the synthesis of complex molecules for various scientific and industrial applications. The intricate balance between electronic arrangement and ligand interaction paves the way for the vast diversity observed in the coordination chemistry landscape.

Geometry Basics
Coordination complexes are at the heart of inorganic chemistry, playing pivotal roles from catalysis in industrial processes to essential biological functions. The spatial arrangement of ligands around the central metal ion in these complexes defines not just their physical and chemical properties but also their applications. Two common geometries observed in coordination complexes are square planar and tetrahedral. Understanding the nuances between these two geometries can provide insights into their behavior and uses.
Square Planar
Definition and Characteristics
The square planar geometry features a central metal ion surrounded by four ligands positioned at the corners of a square. This arrangement results in a planar molecule with the metal at the center, exhibiting 90-degree angles between all ligands. Square planar complexes are typically associated with d^8 metal ions, such as nickel(II), platinum(II), and palladium(II). The configuration is stabilized by the symmetrical distribution of ligands and the metal’s electronic arrangement, favoring metals with a full d^8 electron configuration.
Typical Metal Ions and Ligand Arrangement
In square planar complexes, the selection of metal ions and ligands is crucial for forming stable complexes. Common metals include:
- Platinum(II)
- Palladium(II)
- Nickel(II)
- Gold(III)
These metals form strong bonds with various ligands, including halides, amines, and carbon monoxide, allowing for a diverse range of compounds with significant chemical activity and applications, especially in catalysis and medicine.
Tetrahedral
Definition and Characteristics
Tetrahedral complexes are defined by a central metal ion surrounded by four ligands at the corners of a tetrahedron. This geometry is characterized by ligand-metal-ligand angles of approximately 109.5 degrees, providing a three-dimensional structure. Tetrahedral coordination is common for metal ions with a d^0, d^10, or high spin d^5 configuration, such as zinc(II), magnesium(II), and iron(II). The lack of central symmetry distinguishes tetrahedral complexes from square planar, affecting their physical properties and reactivity.
Common Metal Ions and Ligand Coordination
Metals that typically form tetrahedral complexes include:
- Zinc(II)
- Magnesium(II)
- Iron(II)
These metals interact with a wide range of ligands, from simple anions like chloride and hydroxide to more complex organic molecules. The flexibility in ligand choice and the spatial arrangement of the tetrahedral geometry make these complexes integral in biological systems and materials science.
Key Differences
Coordination Number
- Square Planar: Coordination number of 4, with ligands arranged in a single plane.
- Tetrahedral: Also has a coordination number of 4, but with ligands arranged in a three-dimensional structure.
Angle Between Ligands
- Square Planar: Features 90-degree angles between all ligands.
- Tetrahedral: Ligands are separated by 109.5 degrees, creating a more spread-out structure.
Metal Ion Preferences
Square planar complexes are typically formed by d^8 metals, favoring low-spin configurations. Tetrahedral complexes, on the other hand, are more versatile, accommodating d^0, d^10, and high-spin d^5 configurations, reflecting their adaptability in various chemical environments.
Ligand Field Stabilization
The energy stabilization resulting from the arrangement of ligands around the metal ion differs significantly between the two geometries. Square planar complexes benefit from greater ligand field stabilization energy (LFSE), particularly for d^8 configurations, due to the specific electronic interactions within the d-orbitals. Tetrahedral complexes have lower LFSE, owing to their less symmetrical arrangement and lesser extent of d-orbital splitting.
Color and Chemical Behavior
The difference in ligand arrangement and electronic interactions also influences the color and chemical reactivity of these complexes. Square planar complexes often display vibrant colors and participate in complex reactions, such as ligand substitution and redox processes, useful in catalysis and drug design. Tetrahedral complexes are generally less colored and show distinct reactivity patterns, important in biological processes and the synthesis of materials.
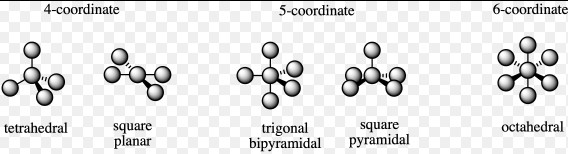
Factors Influencing Geometry
The geometry of coordination complexes is not arbitrary; it’s determined by a variety of factors ranging from the electronic configuration of the central metal ion to the steric demands of the ligands. Understanding these factors can provide insights into the stability and reactivity of complexes.
Electronic Configuration
Impact on Shape Selection
The electronic configuration of the central metal ion is a primary determinant of a complex’s geometry. For instance:
- d^8 configuration often leads to square planar geometries due to the stabilization provided by the arrangement of electrons in the d-orbitals.
- d^0 or d^10 configurations are more likely to form tetrahedral complexes, where there is no need for the additional stabilization square planar geometry offers.
This relationship between electron configuration and geometry helps predict the structure of unknown complexes and understand their electronic properties.
Techniques for Identifying Complex Shapes
Ligand Types and Strength
Role in Determining Complex Geometry
Ligands can dramatically influence the geometry of a coordination complex. Key factors include:
- Field strength: Strong field ligands, such as CN^- or CO, tend to promote lower coordination numbers and more compact geometries like square planar.
- Size and shape: Larger or more bulky ligands may enforce geometries that minimize steric clashes, often leading to tetrahedral configurations.
The interaction between ligand field strength and the metal’s electronic configuration can predict the resulting complex geometry.
Steric Hindrance
Influence on Preferred Structure
Steric hindrance refers to the physical impediment to the optimal arrangement of atoms due to the size of the ligands. This factor is crucial in:
- Limiting the number of ligands that can bind to a central metal ion, affecting the coordination number.
- Influencing the geometry by favoring arrangements that minimize repulsions between large ligands.
Steric effects can override electronic preferences, particularly in complexes with bulky ligands, directing the complex towards a tetrahedral rather than a square planar geometry.

Applications and Examples
The geometry of coordination complexes underpins their utility in a vast range of applications, from industrial catalysis to the development of new pharmaceuticals.
Square Planar Complexes
Role in Catalysis and Pharmaceuticals
Square planar complexes are renowned for their roles in:
- Catalysis: The reactivity and specificity of square planar complexes, such as platinum-based catalysts, are pivotal in many industrial processes, including the synthesis of fine chemicals and pharmaceuticals.
- Pharmaceuticals: Drugs like cisplatin, a square planar platinum complex, are used in chemotherapy treatments, taking advantage of their ability to bind to DNA and disrupt cancer cell growth.
Notable Examples
- Cisplatin (Pt(NH3)2Cl2): A potent chemotherapeutic agent.
- Rhodium catalysts: Used in the hydroformylation of alkenes.
Tetrahedral Complexes
Significance in Biological Systems and Materials Science
Tetrahedral complexes play critical roles in:
- Biological systems: Many enzymes that contain metal ions, such as zinc in carbonic anhydrase, are based on tetrahedral coordination, essential for biochemical reactions.
- Materials science: Tetrahedral complexes are fundamental in the structure of many materials, including semiconductors and zeolites, which have applications in catalysis and gas separation.
Key Examples
- Zn(II) in carbonic anhydrase: Facilitates the rapid conversion of CO2 to bicarbonate and protons, critical in physiological pH buffering.
- Silicon tetrafluoride (SiF4): A tetrahedral molecule used in the production of optical fibers and semiconductors.

Choosing Between Geometries
Determining the geometry of a coordination complex is crucial for predicting its properties and reactivity. Both experimental techniques and theoretical guidelines play roles in this determination.
Predicting Geometry
Guidelines and Considerations
To predict the geometry of a complex:
- Consider the electronic configuration of the metal ion; d^8 configurations lean towards square planar, while d^0 or d^10 favor tetrahedral.
- Assess the ligand field strength; strong field ligands tend to support square planar geometries.
- Evaluate steric hindrance; bulky ligands might push the complex towards a tetrahedral geometry to minimize repulsion.
Experimental Determination
Several experimental techniques can confirm the geometry of a coordination complex:
- X-ray crystallography: Provides detailed information on the arrangement of atoms within a molecule, directly revealing its geometry.
Frequently Asked Questions
What is a coordination complex?
A coordination complex consists of a central metal atom or ion bonded to a surrounding group of molecules or anions, known as ligands. These entities form through coordinate covalent bonds, where both electrons in the bond originate from the ligand. Coordination complexes are pivotal in various chemical processes, including catalysis, electronic devices, and biological systems.
How do square planar and tetrahedral complexes differ in color?
The color of coordination complexes arises from d-d electron transitions and charge transfer between the metal and ligands. Square planar complexes typically exhibit intense colors due to the splitting of d-orbitals in their specific geometry, leading to specific absorbance in the visible spectrum. Tetrahedral complexes, with less orbital splitting, often show different coloration patterns, which can be less intense compared to their square planar counterparts.
Why are some metals more likely to form square planar complexes?
Certain metals, especially those with a d^8 electronic configuration like nickel(II), palladium(II), platinum(II), and gold(III), are more prone to forming square planar complexes. This preference is attributed to the energy stabilization provided by the square planar geometry for these specific electronic configurations, which minimizes repulsion between electrons in the d-orbitals and maximizes the bonding interaction with ligands.
Can a complex switch between tetrahedral and square planar geometries?
Yes, a complex can switch between tetrahedral and square planar geometries under certain conditions, such as changes in ligand concentration, temperature, or pressure. This transformation is significant in catalytic processes and the activation of small molecules where the geometry of the complex can influence its reactivity and selectivity.
Conclusion
The distinction between square planar and tetrahedral complexes is more than just a matter of geometric curiosity; it is a fundamental aspect that influences the reactivity, stability, and color of coordination compounds. This difference underscores the intricate relationship between electronic structure, ligand characteristics, and geometric arrangement, guiding chemists in the synthesis and application of novel materials and molecules.
As we continue to explore the vast domain of coordination chemistry, understanding these geometric nuances offers a lens through which we can better comprehend the complex interactions at play. The ability to predict and manipulate the geometry of coordination complexes opens new avenues for innovation across various fields, from materials science to pharmaceutical development, highlighting the endless potential of chemistry to solve real-world challenges.