The Rankine and Brayton cycles are cornerstones in the field of thermodynamics, playing crucial roles in the generation of power across various industries. These cycles, each with its unique process and application, transform heat into work, driving the turbines that produce electricity. They are fundamental in understanding how power plants and engines operate, making them indispensable to engineers and industry professionals alike.
The Rankine cycle is a steam-based process that utilizes four main steps: heating, evaporating, expanding, and condensing to convert thermal energy into mechanical work. On the other hand, the Brayton cycle operates on a gas, typically air, and follows a continuous process of compression, heating, expansion, and cooling. These differences underscore the distinct efficiencies and applications of each cycle in power generation and propulsion systems.
Within the context of power generation and propulsion, the Rankine and Brayton cycles serve as the backbone for thermal and mechanical engineering applications. Their comparison reveals insights into the efficiency, environmental impact, and technological advancements shaping the future of energy production. By examining the core principles, components, and applications of each cycle, one can appreciate their significance in the modern world and the continuous efforts to enhance their performance.

Rankine Cycle Explained
Basic Concepts and Operation
The Rankine cycle is a thermodynamic process used to convert heat into work, primarily within power generation stations. It consists of four main steps that involve the heating, expansion, condensation, and pumping of water to complete a cycle that efficiently transforms thermal energy into mechanical power. This cycle plays a critical role in steam turbine systems found in nuclear, coal, and thermal solar power plants.
- Heating: Water is heated in a boiler until it becomes high-pressure steam.
- Expansion: This steam expands through a turbine, generating mechanical power.
- Condensation: After expansion, steam is condensed back into water.
- Pumping: Water is then pumped back into the boiler, completing the cycle.
Key Components and Their Functions
- Boiler: Produces high-pressure steam from water.
- Turbine: Converts steam’s thermal energy into mechanical energy.
- Condenser: Cools steam back into water.
- Feedwater Pump: Pumps water back into the boiler.
Each component is crucial for the cycle’s efficiency and functionality, ensuring a continuous process of energy conversion.
Applications and Efficiency
The Rankine cycle is fundamental in power generation, especially where large amounts of electricity are required. It’s efficient in converting heat into work, making it ideal for nuclear, coal-fired, and thermal solar power plants. The cycle’s efficiency can be improved by superheating the steam, reheating it, or increasing the boiler pressure, thereby producing more work per cycle.
Brayton Cycle Overview
Fundamental Principles
The Brayton cycle, also known as the Joule cycle, operates on a continuous process of compression, heating, expansion, and exhaust. It is the basis for gas turbine engines, including jet engines. This cycle utilizes air as its working fluid, different from the Rankine cycle’s use of water.
- Compression: Air is compressed, increasing its pressure.
- Heating: The compressed air is then heated, usually by burning fuel, which significantly increases its volume.
- Expansion: This hot, high-pressure air expands through a turbine, generating power.
- Exhaust: The used air is released into the atmosphere.
Major Elements and Their Roles
- Compressor: Increases the pressure of incoming air.
- Combustor: Heats the compressed air by burning fuel.
- Turbine: Extracts energy from the hot, pressurized air.
- Exhaust: Releases used air and completes the cycle.
Each component’s efficiency directly impacts the overall cycle’s performance, emphasizing the need for advanced materials and technology in Brayton cycle applications.
Usage and Efficiency
The Brayton cycle is widely used in jet propulsion and power generation systems. Its adaptability to different fuel types and high power-to-weight ratio make it suitable for a wide range of applications, from aviation to natural gas power plants. Efforts to improve the Brayton cycle’s efficiency focus on increasing the temperature at which combustion occurs and improving turbine and compressor designs.
Core Differences
Process Flow Comparison
While both cycles aim to convert heat into work, their process flows significantly differ. The Rankine cycle operates in a closed loop, recycling water through phase changes. In contrast, the Brayton cycle is an open loop that continuously intakes and exhausts air without recycling. This fundamental difference affects each cycle’s efficiency, environmental impact, and application suitability.
Efficiency and Output Variations
Efficiency in the Rankine cycle is often enhanced by superheating steam or increasing operational pressure, whereas Brayton cycle efficiency benefits from higher combustion temperatures and compressor-turbine efficiency improvements. Brayton cycles typically exhibit higher power-to-weight ratios, making them ideal for aviation and portable applications, while Rankine cycles are preferred for stationary power generation due to their ability to utilize a wider range of heat sources.
Application Areas
The application areas of these cycles reflect their operational characteristics and efficiencies. The Rankine cycle is predominantly found in stationary power plants where large-scale, efficient power generation is required. On the other hand, the Brayton cycle sees extensive use in aircraft engines and portable power generation units due to its high efficiency and power-to-weight ratio in applications requiring continuous air intake and exhaust.
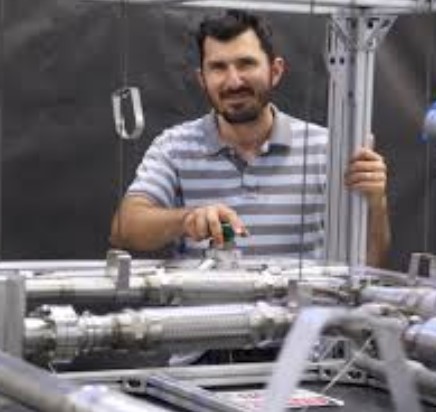
Efficiency Factors
Efficiency in thermodynamic cycles like Rankine and Brayton is pivotal for energy conservation and cost reduction in power generation. It dictates how effectively a cycle converts heat into usable work or electricity. Both cycles have inherent efficiency factors that can be optimized through various enhancements and technological improvements.
Rankine Cycle Efficiency Enhancements
Enhancements in the Rankine cycle aim to maximize the conversion of thermal energy to mechanical work. Key strategies include:
- Superheating Steam: Increasing the temperature of steam beyond its boiling point without increasing pressure, which reduces moisture content and turbine blade erosion.
- Reheating: Passing steam through the turbine in stages, reheating it in between, to maintain a high thermal efficiency over a wider range of operation.
- Regenerative Feedwater Heating: Utilizing steam extracted from the turbine to preheat the boiler feedwater, which increases the average temperature at which heat is added to the cycle.
- Increasing Boiler Pressure: Operating at higher pressures increases the cycle’s thermal efficiency by enabling higher temperatures during heat addition.
Brayton Cycle Efficiency Improvements
For the Brayton cycle, efficiency improvements focus on enhancing the compressor and turbine performance and operating at higher temperatures:
- Advanced Materials: Using materials that can withstand higher temperatures allows for higher combustion temperatures and, consequently, improved cycle efficiency.
- Cooling Technologies for Turbines: Implementing advanced cooling methods in turbines to enable higher operational temperatures without damaging the turbine blades.
- Intercooling: Cooling the air between compression stages to reduce the work required to compress the air further.
- Reheat: Similar to the Rankine cycle, adding heat in stages in the Brayton cycle can improve efficiency by maintaining a higher average temperature during the expansion process.
Comparative Analysis of Efficiency
Comparing the efficiency of Rankine and Brayton cycles involves examining their thermodynamic properties and application-specific considerations. The Rankine cycle generally has higher efficiencies in stationary power plants due to its ability to recover and recycle heat. Meanwhile, the Brayton cycle’s efficiency shines in applications requiring high power-to-weight ratios, such as in jet engines, where its continuous flow process and capacity for high temperatures optimize performance.
Environmental Impact
Emissions and Sustainability
The environmental impact of both cycles is closely tied to their emissions and sustainability. The Rankine cycle, especially in coal-fired power plants, faces challenges due to high CO2 emissions. Innovations in carbon capture and storage (CCS) technologies are critical for reducing these emissions. The Brayton cycle, used in natural gas turbines, typically emits less CO2 per unit of energy produced compared to coal, making it a relatively cleaner option. However, minimizing methane leaks during natural gas extraction is crucial for maximizing its environmental benefits.
Role in Renewable Energy
Both cycles play significant roles in renewable energy. The Rankine cycle is integral to geothermal and solar thermal power plants, converting natural heat into electricity with minimal emissions. The Brayton cycle’s adaptability allows it to integrate with solar power and biofuel sources, reducing reliance on fossil fuels. As renewable energy technologies advance, these cycles’ flexibility and efficiency improvements will be key to their expanded use and integration into the energy mix.
Advancements and Innovations
Recent Technological Advancements
Recent technological advancements have pushed the boundaries of both cycles’ performance and environmental impact. In the Rankine cycle, developments in binary cycle technology and low-temperature evaporators have opened new possibilities for geothermal and waste heat recovery applications. For the Brayton cycle, additive manufacturing is revolutionizing turbine and compressor designs, allowing for more complex geometries that improve airflow and efficiency. Furthermore, hybrid systems that combine both cycles, such as in combined cycle gas turbine (CCGT) power plants, significantly enhance overall efficiency by utilizing waste heat from the Brayton cycle to power a Rankine cycle.
Future Prospects
The future prospects for the Rankine and Brayton cycles are closely tied to global energy demands and the push for sustainable energy solutions. As the world moves towards decarbonization, the emphasis will be on further optimizing these cycles for renewable energy sources, improving their efficiency, and reducing their environmental footprint. Innovations in materials science, thermal engineering, and hybrid systems will play a crucial role in shaping the next generation of power generation technologies. With ongoing research and development, these cycles will continue to evolve, offering more efficient, flexible, and environmentally friendly solutions for global energy needs.
Frequently Asked Questions
What is the Rankine Cycle?
The Rankine cycle is a thermodynamic process used primarily in power generation systems, notably in steam turbines. It involves the conversion of heat into work through a series of phases: heating in a boiler, expansion through a turbine, condensation, and finally, pumping the condensed water back into the boiler. This cycle is pivotal in electricity generation, especially in coal, nuclear, and thermal solar power plants, due to its efficiency in converting thermal energy into mechanical power.
How does the Brayton Cycle work?
The Brayton cycle, also known as the Joule cycle, is the foundational thermodynamic process behind gas turbines and jet engines. It operates by compressing air, heating it through combustion or another heat source, expanding it through a turbine to generate work, and then releasing it. This continuous flow cycle is crucial for its application in aircraft propulsion and power plants, offering the advantages of high power-to-weight ratio and efficiency in converting fuel into kinetic energy.
What are the main differences between Rankine and Brayton cycles?
The primary difference between the Rankine and Brayton cycles lies in their working fluid and operational process. The Rankine cycle uses water/steam and operates in a closed loop, involving phase changes between liquid and vapor. Conversely, the Brayton cycle uses air or other gases and operates on a continuous flow basis without phase changes. Efficiency, application, and environmental impact also vary significantly, with each cycle optimized for specific types of power generation and propulsion systems.
Why is efficiency important in these thermodynamic cycles?
Efficiency in the Rankine and Brayton cycles directly impacts the amount of power generated from a given amount of fuel or heat source. Higher efficiency means more energy conversion into useful work, reducing fuel consumption and decreasing operational costs. It also has a significant environmental impact, as improved efficiency leads to lower emissions of greenhouse gases and pollutants. Therefore, enhancing the efficiency of these cycles is a key focus in research and development, aiming for more sustainable and cost-effective power generation and propulsion technologies.
Conclusion
The Rankine and Brayton cycles are foundational to the generation of power and propulsion, each with its unique mechanisms and contributions to the field of thermodynamics. Their comparison not only highlights the distinct applications and efficiencies inherent to steam and gas-based systems but also underscores the ongoing innovations aimed at enhancing their performance. Understanding these cycles offers valuable insights into the sustainable development and technological advancements in energy production and propulsion, reflecting the dynamic nature of engineering solutions in response to global energy needs.
As the world continues to seek more efficient and environmentally friendly energy sources, the significance of optimizing the Rankine and Brayton cycles cannot be overstated. These cycles represent the intersection of engineering innovation and environmental stewardship, driving the evolution of power generation and propulsion technologies towards a more sustainable future. Their study and improvement remain pivotal in meeting the growing global demand for energy while minimizing the environmental footprint of power generation and transportation.