Kinetic isotope effects play a pivotal role in the intricate world of chemical reactions, serving as a window into the mechanisms that drive them. These effects, arising from the use of isotopically distinct versions of the same chemical element, offer chemists a powerful tool to probe reaction dynamics and energy landscapes. The subtle, yet profound, differences in physical properties between isotopes can significantly impact the rate at which chemical reactions occur, providing invaluable insights into their pathways.
The primary and secondary kinetic isotope effects refer to changes in reaction rates due to the substitution of isotopes in molecules involved in the reaction. The primary effect occurs when the isotope substitution is in a bond that is broken or formed in the rate-determining step of the reaction, typically resulting in a more pronounced change in reaction rate. The secondary effect, though generally less impactful, arises from substitution at a site not directly involved in the rate-determining step, affecting the reaction rate through changes in bond vibrations or electronic structure.
These effects not only underscore the nuanced interplay between atomic mass and chemical reactivity but also equip scientists with the means to elucidate complex reaction mechanisms. By examining how the substitution of isotopes influences reaction rates, researchers can infer details about transition states, energy barriers, and even the role of quantum mechanics in chemical processes.
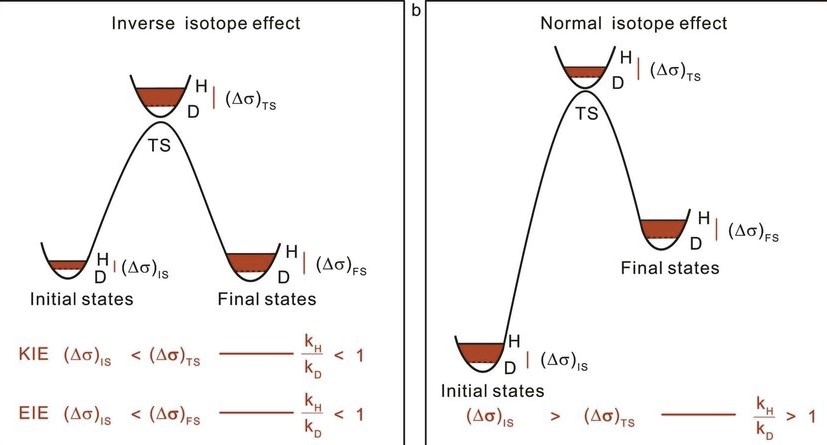
Basic Concepts
Kinetic Isotope Effect
Definition
The Kinetic Isotope Effect (KIE) is a phenomenon observed in chemical reactions where changing one atom for its isotope alters the reaction rate. Isotopes are atoms of the same element with different numbers of neutrons, giving them slightly different physical properties. The KIE shines a light on how these minute differences can significantly impact chemical processes.
Role in Chemistry
The KIE is pivotal for chemists seeking to understand reaction mechanisms. It offers insights into the transition states and energy requirements of reactions. By analyzing how isotopes affect reaction rates, chemists can deduce which bonds are breaking and forming, and at what stage of the reaction these changes occur. This knowledge is crucial for designing more efficient reactions in both research and industrial settings.
Isotopes
What Are Isotopes?
Isotopes are variants of a particular chemical element that have the same number of protons but different numbers of neutrons in their nuclei. This difference in neutron count does not affect the chemical properties of an element in a significant way under normal conditions. However, isotopes have different masses, which can lead to slight variations in their physical and nuclear properties.
Stable vs. Radioactive Isotopes
Isotopes can be broadly classified into two categories: stable and radioactive.
- Stable isotopes do not change under normal conditions. They remain as they are, making them extremely useful in a variety of scientific applications, including the study of metabolic pathways and geological formations.
- Radioactive isotopes, on the other hand, are unstable and decay over time, emitting radiation in the process. These isotopes are invaluable in medical diagnostics, treatment, and nuclear energy production.
Reaction Rate
Explanation
The reaction rate refers to the speed at which reactants are converted into products in a chemical reaction. It’s a measure of the change in concentration of reactants (or products) per unit time. Reaction rates can vary widely, from fractions of a second to years, depending on the nature of the reactants and the conditions under which the reaction occurs.
Factors Affecting Rate
Several factors influence the rate of a reaction, including:
- Temperature: Generally, higher temperatures increase reaction rates.
- Concentration of reactants: More reactants usually mean faster reactions.
- Presence of a catalyst: Catalysts can significantly speed up reactions without being consumed.
- Surface area: In reactions involving solids, more surface area can lead to faster reactions.
- Pressure: For reactions involving gases, higher pressures can increase reaction rates.
Primary Kinetic Isotope Effect
Definition
The Primary Kinetic Isotope Effect is observed when the substitution of an isotope directly impacts a bond that is broken or formed in the rate-determining step of a chemical reaction. This effect can significantly alter the reaction rate because it directly affects the transition state of the reaction.
What It Is
The primary KIE is a powerful tool for understanding chemical reactions at a molecular level. It hinges on the principle that heavier isotopes (like deuterium replacing hydrogen) will form and break bonds slightly differently than their lighter counterparts. This difference is often measurable and can provide valuable insights into the workings of the reaction.
How It Differs
The primary KIE differs from other isotopic effects because it directly influences the rate-determining step of a reaction—the slowest step that determines the overall reaction rate. This direct involvement means that primary KIEs tend to have a larger impact on reaction rates compared to secondary kinetic isotope effects.
Mechanism
Breaking of Bonds
The mechanism behind the primary KIE is rooted in the breaking and forming of chemical bonds involving isotopes. Heavier isotopes lead to a lower vibrational frequency of the bond, which in turn affects the activation energy required for the reaction. The difference in activation energy is what causes the variation in reaction rates.
Impact on Reaction Rate
The impact of the primary KIE on reaction rate can be substantial. Reactions involving heavier isotopes tend to proceed more slowly than those involving their lighter counterparts. This is because the energy barrier for bond breaking/forming is higher when heavier isotopes are involved.
Applications
In Research
In research, the primary KIE is used to:
- Identify the rate-determining step in complex reactions.
- Study enzyme-catalyzed reactions, providing insights into the mechanisms of biological processes.
- Develop and refine theoretical models of chemical reactions.
In Industry
In the industrial context, understanding and utilizing the primary KIE can lead to:
- Improved synthesis routes, optimizing the efficiency and yield of chemical processes.
- Enhanced drug design and development, by elucidating the metabolic pathways of potential pharmaceuticals.
- Better catalyst design, by providing a deeper understanding of how reactions proceed at the molecular level.
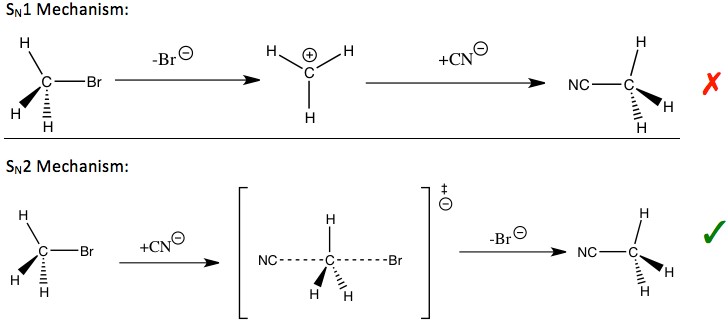
Secondary Kinetic Isotope Effect
Definition
The Secondary Kinetic Isotope Effect (SKIE) refers to the change in reaction rate that occurs when an isotope is substituted at a position not directly involved in the rate-determining step of a chemical reaction. Unlike the primary effect, the SKIE often affects the reaction more subtly, shedding light on the peripheral interactions within the molecular structure.
What It Is
SKIE is a crucial concept in chemical kinetics and mechanism studies. It provides insights into how changes in the mass of isotopes, situated away from the main action site of a reaction, can still influence the overall reaction speed. This effect is particularly valuable for understanding the dynamics of complex molecular systems.
Contrast with Primary
While the primary kinetic isotope effect focuses on isotopes in bonds that break or form in the rate-determining step, the secondary effect involves isotopes in bonds that do not directly participate in this critical step. The impact of SKIE on reaction rates is typically less pronounced than the primary effect but is essential for detailing the subtleties of reaction mechanisms.
Mechanism
Bond Vibration and Reorganization
The mechanism behind SKIE involves alterations in bond vibrations and molecular reorganization. Isotope substitution affects the vibrational modes of a molecule, which can influence how a molecule approaches its transition state during a reaction. These vibrational changes, though secondary, play a crucial role in the energy landscape of the reaction pathway.
Effect on Reaction Kinetics
SKIE can alter reaction kinetics by affecting the distribution of energy within a molecule. This redistribution can either raise or lower the activation energy required for a reaction, thereby changing its rate. Understanding these effects is key to deciphering complex reaction mechanisms where multiple steps and pathways are involved.
Applications
Diagnostic Tool
SKIE serves as a powerful diagnostic tool for chemists and researchers. By observing how isotope substitution influences reaction rates, scientists can:
- Identify subtle aspects of reaction mechanisms.
- Distinguish between competing pathways.
- Probe the involvement of specific bonds and groups in a reaction.
Understanding Complex Reactions
The application of SKIE in studying complex reactions is invaluable. It helps in:
- Clarifying the roles of non-participating groups in a reaction.
- Elucidating the energy distribution within molecules.
- Enhancing the understanding of catalysis and enzyme function.
Comparison
Key Differences
Bond Involvement
- Primary KIE: Involves isotopes in bonds directly participating in the rate-determining step.
- Secondary KIE: Involves isotopes in bonds not directly participating in the rate-determining step.
Reaction Rate Influence
- Primary KIE tends to have a more significant impact on reaction rates due to its direct involvement with the reaction’s critical pathway.
- Secondary KIE generally influences reaction rates to a lesser extent, providing insight into the broader molecular environment.
Similarities
Despite their differences, both primary and secondary KIE share common ground in their:
- Role in determining reaction mechanisms: They are indispensable tools for unraveling the steps and transitions within chemical reactions.
- Use in analytical chemistry: Both effects are employed to refine and confirm theoretical models of reaction dynamics.
Implications
In Chemical Synthesis
Optimization of Reaction Conditions
Understanding both primary and secondary KIE allows chemists to optimize reaction conditions by:
- Tailoring the reaction pathway for better yields.
- Selecting appropriate isotopic labeling for mechanistic studies.
- Minimizing undesirable side reactions.
Isotope Labeling
Isotope labeling is a direct application of KIE, enabling:
- Tracing of specific atoms through a reaction mechanism.
- Study of complex organic and inorganic reaction systems.
- Development of novel synthetic methodologies.
In Biological Systems
Enzyme-catalyzed Reactions
KIEs are crucial for understanding enzyme-catalyzed reactions, offering insights into:
- The specificity of enzyme-substrate interactions.
- The dynamics of enzyme-mediated transformations.
- The roles of non-active site residues in catalysis.
Drug Development
In drug development, KIEs aid in:
- Identifying metabolic pathways for drug molecules.
- Designing more effective and selective drugs.
- Understanding drug-resistance mechanisms.
Challenges and Considerations
Measurement Techniques
The accuracy and precision of measuring KIEs are critical, requiring:
- Advanced spectroscopic and analytical methods.
- Careful experimental design to minimize errors.
- Calibration against known standards for reliability.
Limitations
Despite their utility, KIE studies face limitations, such as:
- The potential for isotopic fractionation.
- The influence of external conditions on reaction rates.
- The need for sophisticated equipment and expertise.
Interpretation of Results
Factors Influencing Interpretation
Interpreting KIE results demands consideration of:
- The complex interplay of forces within molecules.
- The possibility of competing reaction pathways.
- Environmental and experimental variables.
Common Pitfalls
Researchers must navigate common pitfalls, including:
- Overreliance on KIEs without corroborating evidence.
- Misinterpretation of subtle isotope effects.
- Neglecting the influence of solvent and temperature.
Frequently Asked Questions
What is the kinetic isotope effect?
The kinetic isotope effect describes the change in reaction rate observed when one atom in the reactants is replaced by its isotope. This phenomenon is a direct consequence of the differences in mass between isotopes, which leads to variations in vibrational energies and, consequently, reaction rates.
How does the primary kinetic isotope effect differ from the secondary?
The primary kinetic isotope effect manifests when the isotope substitution occurs in a bond that breaks or forms in the rate-determining step of a reaction, significantly affecting the reaction rate. In contrast, the secondary kinetic isotope effect results from substitution at a position not directly involved in the rate-determining step, influencing the reaction through alterations in bond vibrations or electronic configurations, usually with a less pronounced impact on the rate.
Why are kinetic isotope effects important in chemical research?
Kinetic isotope effects are crucial for understanding reaction mechanisms at a molecular level. They provide insights into the dynamics of chemical reactions, including the identification of transition states and the determination of energy barriers. This information is invaluable for the development of new synthetic methodologies, the optimization of reaction conditions, and the design of more efficient catalysts in both industrial and academic research settings.
Can kinetic isotope effects be used in biological research?
Yes, kinetic isotope effects are extensively used in biological research to study enzyme-catalyzed reactions. By substituting isotopes within substrates or cofactors, researchers can gain detailed insights into the mechanism of enzyme action, including the identification of catalytic steps, the role of enzyme-substrate interactions, and the factors influencing reaction rates. This approach has profound implications for understanding metabolism, drug action, and the evolution of biological pathways.
Conclusion
The exploration of primary and secondary kinetic isotope effects provides a fascinating glimpse into the realm of chemical and biological reactions, revealing the intricate dance of atoms and molecules that underpins the physical world. These effects serve as critical tools for scientists, enabling the dissection of complex reaction mechanisms and the refinement of theoretical models. Through the careful study of how isotopic variation influences reaction rates, we can unlock deeper understandings of chemical reactivity and catalysis, paving the way for advances in synthesis, drug development, and beyond.
The importance of kinetic isotope effects extends far beyond the confines of academic curiosity, touching on the foundational aspects of chemistry and biology. As researchers continue to harness this powerful analytical technique, the boundaries of our knowledge will expand, leading to new discoveries and innovations that will shape the future of science and technology.