Neutron interactions within the nucleus of an atom represent a cornerstone of nuclear physics, playing a pivotal role in the alchemy of elements and the harnessing of nuclear energy. These interactions, particularly neutron capture and absorption, serve as the bedrock for understanding atomic reactions and their extensive applications, from medical treatments to energy production. The precise nature of these interactions dictates the behavior of atomic nuclei under various conditions, influencing both natural phenomena and human-engineered processes.
Neutron capture occurs when a neutron enters an atomic nucleus, combining with it to form a heavier isotope of the same element. On the other hand, neutron absorption involves a neutron being absorbed by a nucleus, which may lead to different nuclear reactions, including the possibility of causing the nucleus to become unstable and decay. While both processes involve the interaction of neutrons with atomic nuclei, their outcomes and implications differ significantly.
In exploring these nuclear phenomena, we delve into the subtleties that distinguish neutron capture from absorption. These distinctions are not merely academic but have practical consequences in fields ranging from nuclear energy to cancer treatment. Understanding the nuances of neutron interactions aids in the development of technologies for energy generation, medical diagnostics, and beyond, reflecting the profound impact of these atomic-scale events on our macroscopic world.
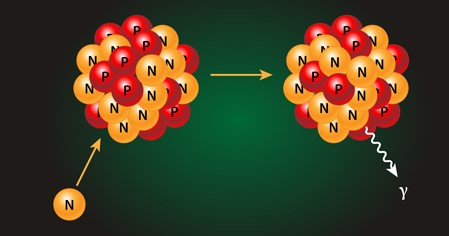
Basic Concepts
Atomic Structure
Atoms form the foundation of all matter in the universe. At the center of each atom lies the nucleus, a dense core made up of protons and neutrons. Protons carry a positive electric charge, while neutrons are neutral, having no charge. The number of protons in the nucleus defines the chemical element (e.g., hydrogen, carbon), and the number of neutrons can vary within atoms of the same element, resulting in different isotopes.
Neutrons: Role and Properties
Neutrons play a critical role in the stability of atoms. Without neutrons, protons in the nucleus would repel each other due to their positive charge. Neutrons act as a buffer, reducing the electrostatic forces of repulsion between protons. Their neutral charge and ability to penetrate other atoms without being repelled make neutrons key players in nuclear reactions.
Nuclear Reactions
Nuclear reactions involve changes in an atom’s nucleus and can lead to the transformation of one element into another. These reactions are fundamental to energy production, medical imaging, and the synthesis of new elements.
Overview of Reactions
Nuclear reactions can release or absorb significant amounts of energy. They include fission (splitting of a nucleus), fusion (combining of nuclei), and radioactive decay. Neutrons are often released during these processes, serving both as products and initiators of further reactions.
Relevance of Neutrons
Neutrons are indispensable in nuclear reactions for several reasons:
- Initiators: In nuclear fission, for instance, a neutron collides with a heavy nucleus, causing it to split and release more neutrons.
- Catalysts: In fusion, neutrons can carry away excess energy, allowing the reaction to continue.
- Probes: Due to their neutral charge, neutrons can penetrate materials without being deflected, making them ideal for imaging and analysis.
Neutron Interactions
Types of Interactions
Neutrons can interact with atomic nuclei in various ways, leading to different types of nuclear reactions. These include elastic scattering, where the neutron bounces off the nucleus without changing it, and inelastic scattering, where the neutron causes the nucleus to become excited, emitting radiation as it returns to stability.
Neutron Capture
Neutron capture is a process where a neutron is absorbed by a nucleus, forming a heavier isotope of the same element. This reaction is crucial in both natural and artificial environments, contributing to the creation of elements in stars and the operation of nuclear reactors.
Process and Outcomes
When a neutron is captured by a nucleus, the compound nucleus becomes excited and usually releases gamma radiation to shed the excess energy. The resulting nucleus is often stable, but in some cases, it can be radioactive, leading to further decay.
Applications
Neutron capture has important applications in:
- Energy production: In nuclear reactors, neutron capture reactions help sustain the fission chain reaction.
- Medical treatments: Boron neutron capture therapy uses captured neutrons to target and destroy cancer cells.
- Scientific research: Neutron capture cross-section measurements provide insights into nuclear structure and processes.
Neutron Absorption
Neutron absorption involves a neutron being fully integrated into the nucleus, which may lead to various outcomes including the nucleus entering a higher energy state or undergoing transmutation into a different element.
Differences from Capture
While neutron capture results in the formation of a heavier isotope of the same element, neutron absorption can change the very nature of the nucleus, leading to the creation of entirely new elements or isotopes.
Results and Implications
Neutron absorption can lead to:
- Transmutation: The transformation of one element into another, which is fundamental in the synthesis of new elements.
- Radioactivity: The absorbed neutron may render the nucleus unstable, leading to radioactive decay.
Industrial Uses
Industries rely on neutron absorption for:
- Radioisotope production: Essential for medical diagnostics and treatments.
- Nuclear waste management: Transmutation of long-lived isotopes into shorter-lived or stable ones.
Comparison
Process Differences
Neutron capture involves a neutron joining with a nucleus to form a heavier isotope, typically releasing gamma rays. Neutron absorption, while similar, can initiate a series of nuclear reactions, leading to the nucleus’s transformation or decay.
Mechanism of Capture vs. Absorption
- Capture: A neutron is added to the nucleus, increasing its mass.
- Absorption: A neutron is absorbed, potentially altering the nucleus’s composition and leading to different nuclear processes.
Energy Considerations
Energy plays a crucial role in determining whether a neutron will be captured or absorbed. Low-energy (thermal) neutrons are more likely to be captured, while high-energy neutrons are more prone to cause other reactions, including absorption leading to fission in certain nuclei.
Outcome Variations
The outcomes of neutron capture and absorption are significantly different, impacting both the immediate environment and broader applications. Capture generally results in a stable isotope, while absorption can lead to a variety of results, from stable isotopes to radioactive decay.
Product Nuclei Characteristics
- Capture: Produces a heavier isotope of the element.
- Absorption: Can change the atomic number, leading to a different element or radioactive isotope.
Applications Contrast
Energy Production
- Capture: Used in control rods to manage reactor activity.
- Absorption: Critical in the fission process for energy release.
Medical Applications
- Capture: Boron neutron capture therapy targets cancer cells specifically.
- Absorption: Produces radioisotopes for diagnosis and treatment.
Research and Development
Both processes are used to study nuclear properties and develop new technologies for material science, energy, and medicine.
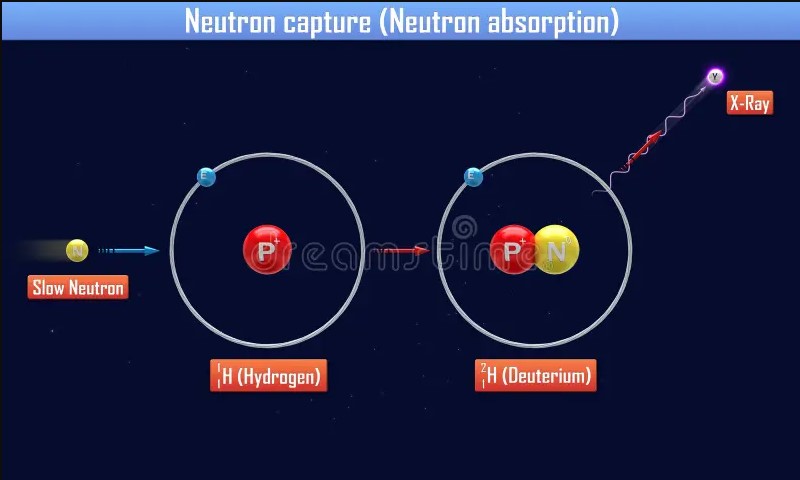
Factors Influencing Interactions
Energy Levels
Thermal vs. Fast Neutrons
Neutrons can be classified based on their energy levels into thermal and fast neutrons. Thermal neutrons are slow-moving and have low energy, making them more likely to be captured by nuclei due to their increased interaction time. Fast neutrons, on the other hand, have high energy and move rapidly, leading to different interaction dynamics, such as higher chances of causing fission in certain isotopes.
Impact on Capture and Absorption
The energy level of a neutron significantly affects its probability of being captured or absorbed by a nucleus. Thermal neutrons are more likely to undergo capture, contributing to processes like the neutron capture therapy in medical applications. Fast neutrons, with their greater energy, are more prone to absorption that results in nuclear fission, a principle harnessed in nuclear power generation.
Material Composition
Isotopic Abundance
The composition of a material, particularly its isotopic abundance, plays a crucial role in neutron interactions. Isotopes of the same element can have vastly different neutron cross-sections, affecting how likely they are to capture or absorb neutrons. For instance, the isotope Boron-10 has a high neutron capture cross-section, making it useful in neutron capture therapy.
Cross-section Variability
The neutron cross-section of an isotope indicates the likelihood of a neutron interacting with it. This variability is crucial in designing nuclear reactors and other systems that rely on controlled neutron interactions. Materials with high cross-sections are often used in reactor control rods to absorb excess neutrons and regulate the reactor’s fission rate.
Significance in Various Fields
Nuclear Energy
Reactor Design and Safety
Neutron interactions are fundamental to reactor design and safety. Control rods, made of materials with high neutron absorption capacities, are essential for regulating the neutron flux and, by extension, the rate of fission reactions. This control mechanism is vital for preventing reactor meltdowns and ensuring the safe production of nuclear energy.
Fuel Cycle Efficiency
Neutron capture plays a significant role in fuel cycle efficiency. It can transmute fertile materials into fissile isotopes, extending fuel life and reducing waste. Understanding and optimizing these interactions can lead to more sustainable and efficient nuclear power generation.
Medical Applications
Cancer Treatment
In cancer treatment, particularly through neutron capture therapy, the choice of isotope and its interaction with neutrons are crucial. Targeted delivery of neutrons to cancerous cells, where they are captured by boron-10 or another suitable isotope, allows for precise destruction of tumors with minimal damage to surrounding healthy tissue.
Diagnostic Techniques
Neutron absorption is used in various diagnostic techniques, including neutron imaging. This method provides insights into materials and biological samples, offering advantages over traditional X-ray imaging by revealing hydrogen-rich areas and other specific features more clearly.
Scientific Research
Neutron Sources
Neutron sources are vital for research in physics, chemistry, and biology. Facilities like spallation sources and nuclear reactors produce neutrons for experiments that probe the structure and properties of materials, helping scientists discover new phenomena and develop innovative technologies.
Material Science Investigations
In material science, neutrons are used to study the arrangement of atoms and the forces between them. These investigations can lead to the development of new materials with desired properties for various applications, from aerospace to electronics.
Challenges and Developments
Technological Limitations
Detection and Measurement Difficulties
One of the main challenges in neutron science is the detection and measurement of neutrons, especially thermal neutrons. Their neutral charge means they do not ionize materials directly, making them harder to detect than charged particles. Advances in detection technology are essential for improving the accuracy and efficiency of neutron-based research and applications.
Advancements in Neutron Technology
Despite these challenges, significant advancements have been made in neutron technology. Innovations in detector materials, neutron generation, and moderation techniques have enhanced our ability to harness neutrons for various purposes, from energy production to the diagnosis and treatment of diseases.
Environmental and Safety Considerations
Radioactive Waste Management
Radioactive waste management is a critical issue in fields that rely on neutron interactions, especially nuclear energy. Developing methods to minimize, recycle, or safely dispose of radioactive waste is crucial for the sustainability and acceptance of nuclear technologies.
Radiation Protection Measures
Radiation protection measures are essential to safeguard researchers, medical personnel, and the public from the potential hazards of neutron radiation. Effective shielding, proper handling protocols, and safety training are necessary to prevent exposure and ensure the safe use of neutron technologies.
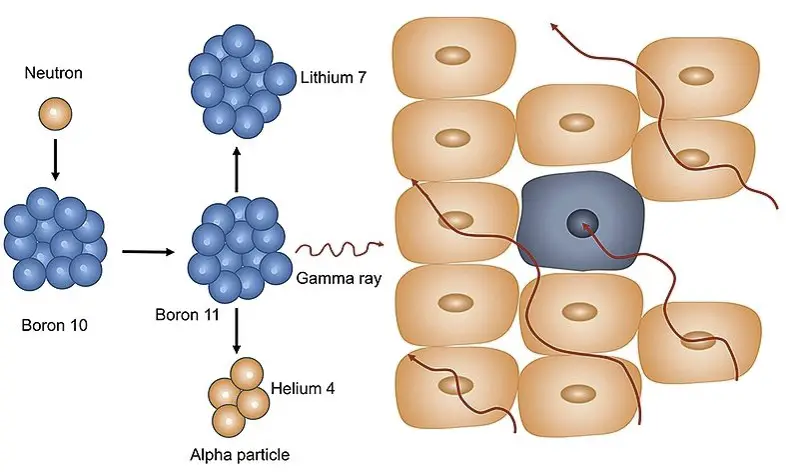
Frequently Asked Questions
What is neutron capture?
Neutron capture is a nuclear reaction where an atomic nucleus absorbs a neutron, becoming a heavier isotope of the same element. This process is crucial for the nucleosynthesis of heavy elements in stars and has applications in nuclear reactors and medical isotopes production.
How does neutron absorption differ from capture?
Neutron absorption refers to the process by which a neutron is absorbed by a nucleus, potentially leading to a variety of outcomes, including the production of a different element or the initiation of a nuclear decay process. It differs from neutron capture, which specifically results in the formation of a heavier isotope of the same element without changing its elemental identity.
Why is understanding neutron interactions important?
Understanding neutron interactions is essential for advancements in nuclear energy, where controlling these reactions is key to reactor design and safety. It also plays a significant role in medical fields, particularly in treatments like neutron capture therapy for cancer, and in scientific research involving material properties and nuclear physics.
Conclusion
The interplay between neutron capture and absorption embodies a fundamental aspect of nuclear science, with wide-ranging implications that extend beyond the confines of theoretical physics into practical applications in energy, medicine, and technology. These interactions not only fuel stars but also empower humanity’s quest for sustainable energy solutions and innovative treatments for diseases.
As research in nuclear physics progresses, the potential to harness these atomic processes more efficiently and safely offers a promising horizon for future developments. By deepening our understanding of neutron interactions, we stand on the cusp of unlocking new capabilities and addressing some of the most pressing challenges facing the world today, demonstrating the profound significance of these microscopic events in shaping our macroscopic reality.