The distinction between the Lyman and Balmer series lies at the heart of quantum mechanics and plays a pivotal role in our understanding of atomic physics. These series, both discovered in the early 20th century, describe the spectral emission lines of the hydrogen atom, which occur when an electron transitions between energy levels. Their discovery was fundamental in the development of quantum theory, shedding light on the quantized nature of energy in atomic systems.
The Lyman series comprises spectral lines in the ultraviolet region, originating from transitions to the n=1 energy level from higher levels. Conversely, the Balmer series consists of lines in the visible spectrum, resulting from transitions to the n=2 energy level. These distinctions are not just academic; they have practical applications in astrophysics, plasma diagnostics, and remote sensing, among other fields.
Understanding these series involves delving into the structure of the hydrogen atom, the simplest of all atoms, which has been a cornerstone in the study of quantum mechanics. The differences between the Lyman and Balmer series reflect the fundamental principles of electron transitions and energy level interactions within atoms, showcasing the diverse ways in which hydrogen can emit or absorb energy.
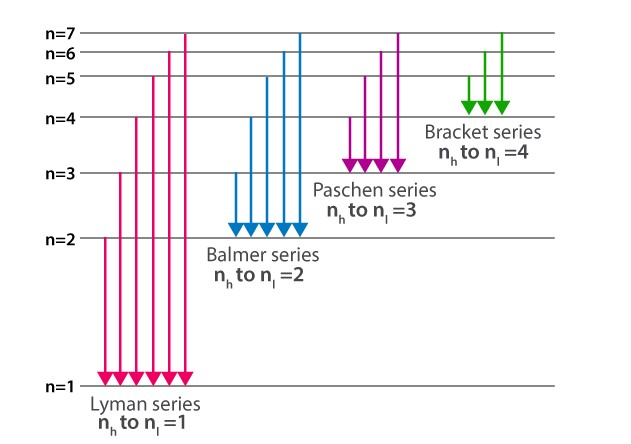
Hydrogen Spectrum Basics
Atomic Structure
The hydrogen atom, often hailed as the simplest of all atoms, consists of just one proton in its nucleus and a single electron bound to the nucleus by electromagnetic force. This simplicity makes it a fundamental model for understanding atomic physics. The behavior of the electron within the hydrogen atom, particularly its transitions between energy levels, lays the groundwork for quantum mechanics and the study of spectral lines.
Hydrogen Atom Simplicity
The simplicity of the hydrogen atom is deceptive, for within this simple structure lies the key to understanding more complex atoms. Hydrogen’s single electron can occupy various energy levels, each characterized by a unique quantum state. It is the movement of this electron between these states that generates the spectral lines we observe in hydrogen’s spectrum.
Electron Transitions
Electron transitions are changes in the energy state of an electron within an atom. When an electron moves from a higher energy level to a lower one, it emits a photon of light. The energy of this photon corresponds to the difference in energy between the two levels. These transitions are quantized, meaning the electron can only occupy specific energy levels.
Spectral Lines
Definition and Significance
Spectral lines are the result of the interaction between electromagnetic radiation and matter. Each line in a spectrum corresponds to a specific photon energy emitted or absorbed as electrons transition between energy levels. The study of these lines, known as spectroscopy, is crucial for understanding atomic and molecular structures.
How They are Observed
Spectral lines are observed using spectroscopes, which disperse light into its component wavelengths. By examining the wavelengths at which light is emitted or absorbed, scientists can determine the energy levels of the electrons in an atom, leading to insights into the atom’s structure and the identification of elements in distant stars and galaxies.
Lyman Series Overview
Discovery and History
In 1906, Johann Lyman, an American physicist, discovered the spectral series of hydrogen now known as the Lyman series. His work revealed a series of ultraviolet emission lines that were not visible to the naked eye, expanding our understanding of the hydrogen spectrum.
Johann Lyman’s Contribution
Lyman’s contribution was monumental. He showed that the ultraviolet lines he observed were due to electron transitions to the n=1 energy level from higher levels. This was the first time such transitions had been observed, and it opened up new avenues in the study of atomic spectra and quantum mechanics.
Wavelength Range
The Lyman series falls entirely within the ultraviolet region of the electromagnetic spectrum. This series includes wavelengths that range from about 91 nm to 121 nm, making them invisible to the human eye but detectable with ultraviolet spectroscopy techniques.
Ultraviolet Region Specifics
The ultraviolet region is significant because it interacts strongly with matter, causing electronic transitions that are crucial for understanding atomic and molecular structures. The ability to observe these transitions in the ultraviolet range is essential for fields such as astrophysics and plasma physics.
Energy Levels
The Lyman series involves electron transitions to the n=1 level from higher energy states. This “ground state” transition is fundamental to the series and distinguishes it from other spectral series of hydrogen.
n=1 Transitions
Transitions to the n=1 level are of high energy, resulting in the emission of ultraviolet photons. These transitions provide insights into the high-energy processes occurring in stars and other celestial bodies.
Applications
Astrophysics and Plasma Diagnostics
The Lyman series has profound applications in astrophysics and plasma diagnostics. In astrophysics, it helps in the study of star formation and the interstellar medium. In plasma diagnostics, the series is used to measure temperature and density in plasma environments, such as those found in fusion reactors.
Balmer Series Overview
Discovery and History
In 1885, Johann Balmer, a Swiss mathematician, empirically discovered a formula to calculate the wavelengths of the visible spectral lines of hydrogen. This discovery of the Balmer series marked a significant step forward in atomic physics, linking mathematics to the quantum behavior of atoms.
Johann Balmer’s Formula
Balmer’s formula predicted the wavelengths of the visible transitions of hydrogen with remarkable accuracy. It was the first time such a relationship had been formulated, demonstrating that the spectral lines followed a predictable pattern, which was a key insight for the development of quantum mechanics.
Wavelength Range
The Balmer series is characterized by electron transitions to the n=2 energy level from higher levels, producing spectral lines in the visible light range. This series includes wavelengths from about 364.6 nm to 656.3 nm, covering colors from deep blue to red.
Visible Light Specifics
The visibility of the Balmer series to the human eye has made it especially important for the field of astronomy. It allows for the direct observation of hydrogen in stellar spectra, providing a window into the composition and conditions of stars and galaxies.
Energy Levels
In the Balmer series, electrons transition to the n=2 level from higher energy states. These transitions produce photons in the visible spectrum, which are key to understanding the lower-energy processes in hydrogen atoms.
n=2 Transitions
n=2 transitions are essential for studying environments where hydrogen is not highly excited, such as cooler stellar atmospheres. They provide critical information about the physical conditions within stars and the interstellar medium.
Applications
Astronomy and Remote Sensing
The Balmer series has extensive applications in astronomy and remote sensing. It is used to identify hydrogen in celestial objects, contributing to our understanding of the universe’s structure and evolution. In remote sensing, the Balmer series helps in analyzing the Earth’s atmosphere and detecting environmental phenomena.
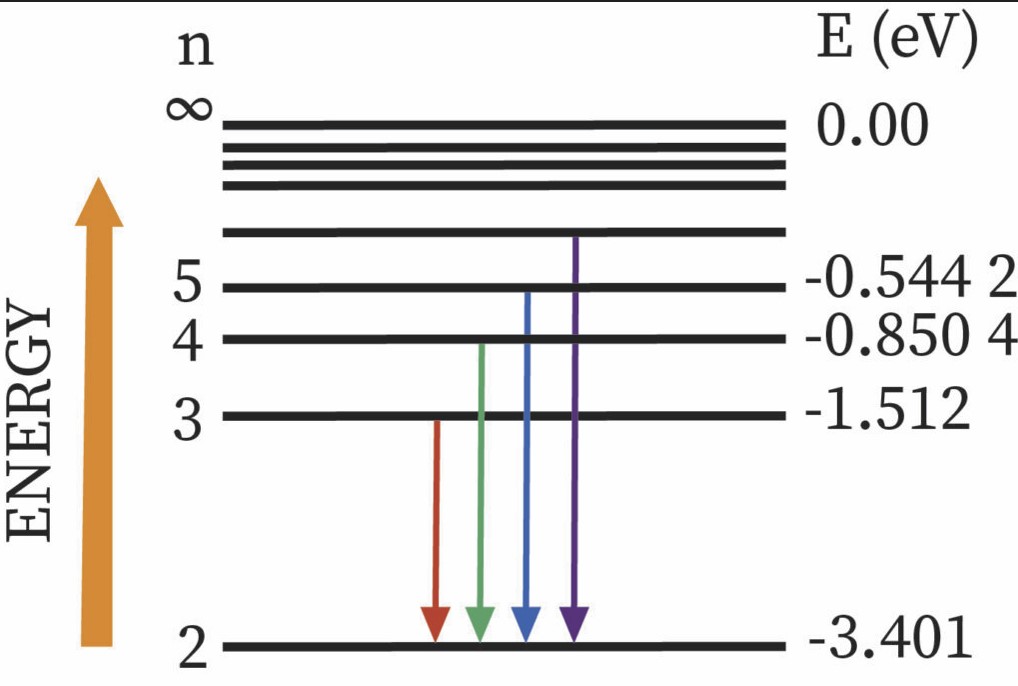
Key Differences
Transition Origins
The Lyman and Balmer series originate from electron transitions within the hydrogen atom, but they differ fundamentally in their initial energy levels. This difference is crucial for understanding the distinct spectral lines they produce.
Initial Energy Levels Compared
- Lyman Series: Transitions to n=1, the lowest energy level.
- Balmer Series: Transitions to n=2, the second-lowest energy level.
These variations in starting points lead to the emission of photons of different energies, thus producing spectral lines in different regions of the electromagnetic spectrum.
Spectral Regions
Ultraviolet vs. Visible Light
- Lyman Series: Falls in the ultraviolet (UV) region, which is invisible to the naked eye but crucial for many scientific applications.
- Balmer Series: Located in the visible light spectrum, easily observed without specialized equipment.
This distinction between ultraviolet and visible light is significant, affecting how these series are used in practical applications and research.
Practical Uses
Distinct Applications in Science and Technology
The Lyman and Balmer series have unique applications owing to their differences in spectral regions and transition origins.
- Lyman Series: Essential in astrophysics for studying high-energy processes and plasma diagnostics in controlled fusion experiments.
- Balmer Series: Crucial for astronomy, particularly in identifying hydrogen in stars and galaxies, and in remote sensing to study the Earth’s atmosphere.
These applications highlight the importance of understanding both series for advancing knowledge in various scientific fields.
Significance in Science
Quantum Mechanics
Role in the Development of Quantum Theory
The discovery and study of the Lyman and Balmer series were instrumental in the development of quantum mechanics. Their predictable patterns of spectral lines provided evidence that energy levels in atoms are quantized, not continuous. This was a radical departure from classical physics and helped to establish the foundation of quantum theory.
- Quantization of Energy: The basis for modern quantum mechanics.
- Spectral Analysis: Provided a method to test and refine quantum theories.
Astronomical Applications
Identifying Stellar Compositions
The Lyman and Balmer series play a pivotal role in astronomy, particularly in the spectroscopic analysis of stars and galaxies. By observing the spectral lines from these series, astronomers can determine the presence and abundance of hydrogen, the most common element in the universe.
- Star Temperatures: Different series can indicate the temperature of stars.
- Galactic Chemistry: Helps in understanding the chemical composition of galaxies.
These insights are crucial for studying the lifecycle of stars and the evolution of galaxies.
Technological Impacts
Spectroscopy Advancements
The study of the Lyman and Balmer series has led to significant advancements in spectroscopy, a field that encompasses a wide range of technologies used in scientific research and industrial applications.
- Instrument Development: Innovations in UV and visible light spectroscopy.
- Analytical Techniques: Enhanced methods for analyzing materials and chemical compositions.
These advancements have applications in medicine, environmental science, and materials engineering, showcasing the broad impact of spectroscopic studies on technology and society.
Frequently Asked Questions
What is the Lyman Series?
The Lyman series refers to the set of spectral emission lines that appear when an electron in a hydrogen atom falls from higher energy levels to the lowest energy level (n=1). These lines are predominantly in the ultraviolet region of the electromagnetic spectrum and are crucial for studying astronomical objects and plasma conditions.
What is the Balmer Series?
The Balmer series consists of spectral lines produced by hydrogen atoms when electrons fall from higher energy levels to the second-lowest energy level (n=2). Visible to the naked eye, these lines have been instrumental in the development of observational astronomy, enabling scientists to identify hydrogen in stars and galaxies.
Why are the Lyman and Balmer series important in astronomy?
These series are vital in astronomy for identifying the presence of hydrogen, the most abundant element in the universe. By analyzing the light from stars and galaxies for the distinct signatures of the Lyman and Balmer series, astronomers can infer the composition, temperature, and density of celestial bodies, contributing to our understanding of stellar life cycles and the interstellar medium.
How do the Lyman and Balmer series differ?
The primary difference between the Lyman and Balmer series lies in their spectral regions and the transitions that cause them. The Lyman series results from electron transitions to the n=1 level and is in the ultraviolet spectrum, whereas the Balmer series arises from transitions to the n=2 level and is visible. This difference is fundamental to their applications in scientific research and technology.
Conclusion
The Lyman and Balmer series, integral to the study of atomic physics, offer profound insights into the behavior of electrons within atoms and the quantized nature of energy. Their discovery not only marked significant milestones in the field of quantum mechanics but also paved the way for numerous applications in modern science and technology. These spectral lines continue to guide researchers in exploring the mysteries of the universe, from the composition of distant stars to the fundamental principles of light and matter.
Understanding the differences between these series enhances our comprehension of the universe’s basic building blocks and their interactions. As we continue to explore the cosmos and delve into the atomic realm, the Lyman and Balmer series remain key to unlocking the secrets of quantum mechanics and its implications for the physical world.