The Hall Effect and the Quantum Hall Effect represent two pivotal discoveries in the field of physics, each providing insights into electrical phenomena and quantum mechanics. The Hall Effect, discovered in 1879 by Edwin Hall, opened the door to new ways of measuring and understanding electrical currents in conductors. In contrast, the Quantum Hall Effect, observed a century later, offers a deeper dive into quantum mechanics by exploring the behavior of electrons in a two-dimensional plane under strong magnetic fields.
The difference between the Hall Effect and the Quantum Hall Effect lies in their fundamental phenomena and applications. The Hall Effect occurs when a magnetic field is applied perpendicular to the flow of electrons in a conductor, creating a voltage across the conductor. This effect is used in various sensing devices. The Quantum Hall Effect, however, is observed in two-dimensional electron systems at very low temperatures and under strong magnetic fields, showcasing quantized Hall resistance.
The importance of these effects extends beyond theoretical physics, influencing practical applications in technology and materials science. The Quantum Hall Effect, for instance, has provided a new standard for electrical resistance based on fundamental constants, with implications for improving measurement precision in various scientific and industrial applications.
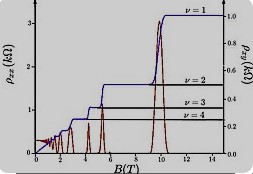
Hall Effect Basics
Definition and Discovery
The Hall Effect is a fundamental principle observed in physics and engineering, which occurs when a magnetic field is applied perpendicular to the flow of electric current in a conductor. This results in the generation of a voltage across the conductor, perpendicular to both the current and the magnetic field. The discovery of the Hall Effect dates back to 1879 when American physicist Edwin Herbert Hall first observed this phenomenon while working on his doctoral thesis at Johns Hopkins University.
How It Works
The working principle of the Hall Effect is straightforward and can be explained in a few simple steps:
- Electric Current Application: An electric current is applied along a strip of conducting material.
- Magnetic Field Introduction: A magnetic field is applied perpendicular to the direction of the electric current.
- Voltage Generation: Due to the Lorentz force, electrons in the conductor are deflected to one side, creating a voltage across the conductor. This voltage is known as the Hall voltage.
This process is critical in understanding how the movement of charges in a conductor can be influenced by external magnetic fields, revealing the charge carrier density and the nature of the charge carriers themselves.
Applications in Technology
The Hall Effect has a wide range of applications in modern technology:
- Magnetic Sensing: Devices such as Hall effect sensors are used to measure the magnitude and direction of magnetic fields.
- Position Sensing: These sensors are also employed in automotive and industrial applications to determine the position of moving parts.
- Current Measurement: Hall effect devices can measure current without direct electrical contact, useful in power monitoring systems.
Quantum Hall Effect Explained
Discovery and Origin
The Quantum Hall Effect was first observed in 1980 by Klaus von Klitzing while experimenting with semiconductor heterostructures at very low temperatures and under strong magnetic fields. This discovery, which expanded on the basic principles of the Hall Effect, earned von Klitzing the Nobel Prize in Physics in 1985. The Quantum Hall Effect is recognized for quantizing the Hall resistance, which means the resistance is observed at certain fixed values.
Core Principles
The Quantum Hall Effect is characterized by the quantization of the Hall conductance. In simple terms, the conductance between the edges of a two-dimensional electron gas exhibits discrete values, which are integer multiples of a fundamental constant. This quantization is remarkably precise and independent of the material details, depending only on fundamental constants.
Quantum Mechanics Involved
The phenomenon involves several complex quantum mechanics principles:
- Landau Levels: Electrons in a magnetic field can only occupy discrete energy levels.
- Edge States: At the boundaries of the conductor, electrons move in one direction, leading to a unidirectional flow that is resistant to scattering.
- Topological Properties: The stability and quantization of the Hall conductance are related to the topological characteristics of the electronic states.
Key Differences
Physical Phenomena
The Hall Effect and Quantum Hall Effect, while based on similar physical principles, exhibit key differences in their phenomena:
- The Hall Effect is an outcome of the classical behavior of electrons under magnetic fields, suitable for macroscopic observation.
- The Quantum Hall Effect, on the other hand, involves quantum mechanical behaviors and is observable only under specific conditions such as extremely low temperatures and high magnetic fields.
Temperature Dependency
Temperature plays a crucial role in differentiating these two effects:
- The classical Hall Effect can be observed at any temperature as long as a magnetic field and a conductor are present.
- The Quantum Hall Effect requires near absolute zero temperatures to minimize the thermal energy that would otherwise disrupt the discrete quantum states.
Material Requirements
The materials used for observing these effects also differ significantly:
- The Hall Effect can be observed in any conductive material.
- The Quantum Hall Effect requires a two-dimensional electron gas typically found in high-purity semiconductor heterostructures.
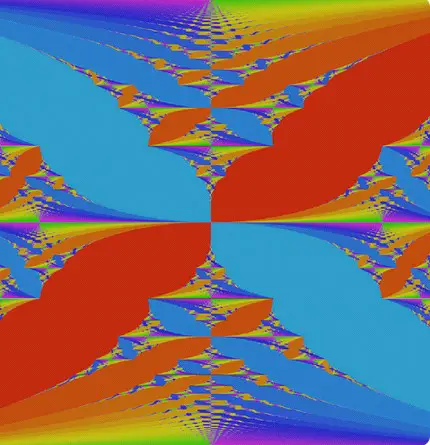
Practical Implications
Use in Electronic Devices
The Hall Effect and Quantum Hall Effect have significantly influenced the development of electronic devices. Hall sensors, developed from the basic principles of the Hall Effect, are integral components in modern electronics, offering both reliability and precision.
- Smartphones and Tablets: These sensors enable features like screen auto-rotation and are crucial for compass functionalities.
- Automotive Systems: Hall sensors detect the position of gears and seats, improving safety and functionality in vehicles.
- Consumer Electronics: Used in everything from washing machines to digital cameras, these sensors help in the precise operation of various devices.
Impact on Sensor Technology
The impact of these phenomena on sensor technology is profound, enhancing the sensitivity and accuracy of sensors used across various industries:
- Magnetic Field Sensing: Hall Effect sensors are used to detect and measure magnetic fields, crucial in geophysical applications.
- Proximity Sensing: These sensors can detect the presence of objects without physical contact, useful in industrial automation.
Contributions to Quantum Computing
The Quantum Hall Effect also plays a pivotal role in the emerging field of quantum computing:
- Topological Quantum Computers: Leveraging the stable quantum states that arise from the Quantum Hall Effect can lead to more robust quantum bits (qubits), which are less susceptible to decoherence.
- Quantum Error Correction: The inherent error-resisting properties of Quantum Hall states help in developing error correction schemes in quantum computing systems.
Theoretical Insights
Theoretical Significance
The theoretical significance of the Quantum Hall Effect cannot be overstated, as it provides a practical method to measure electrical resistance with high precision and repeatability, based on fundamental physical constants.
- Standard Model Testing: The precise quantization of the Hall resistance provides a way to test the accuracy of quantum electrodynamics, a cornerstone of the Standard Model of particle physics.
Influence on Modern Physics
The implications of the Quantum Hall Effect on modern physics are extensive, influencing various theoretical and practical aspects of the field:
- Quantum Field Theory: It has offered insights into gauge invariance and topological field theories.
- Condensed Matter Physics: It has deepened understanding of electron interactions in a low-dimensional system.
Future Research Directions
Ongoing and future research in Hall effects is expected to uncover even more applications and refine existing technologies:
- New Materials: Research into graphene and other two-dimensional materials could yield even more efficient Hall effect sensors.
- Precision Metrology: Improvements in measurement techniques could further enhance the utility of the Quantum Hall Effect in standards and metrology.
Challenges and Limitations
Technical Challenges
Despite their advantages, both effects face technical challenges in application:
- Sensitivity: Enhancing the sensitivity of Hall effect sensors without increasing their cost is a significant challenge.
- Quantum Stability: Maintaining stable quantum conditions for the Quantum Hall Effect outside of laboratory settings remains difficult.
Material and Temperature Constraints
Material science plays a crucial role in the practical application of these phenomena:
- Material Quality: High-purity materials are essential for observing the Quantum Hall Effect, often making it expensive and challenging to implement.
- Temperature Control: The necessity for low temperatures for observing the Quantum Hall Effect limits its applicability in everyday technology.
Limitations in Application
Finally, while promising, the applications of the Hall and Quantum Hall Effects are not limitless:
- Commercial Viability: The cost of implementing quantum technology in commercial applications remains prohibitive.
- Environmental Sensitivity: Hall sensors can be sensitive to environmental factors like temperature and physical stress, which can affect their accuracy.
Frequently Asked Questions
What is the Hall Effect?
The Hall Effect refers to the generation of a voltage across an electrical conductor, transverse to an electric current in the conductor and a magnetic field perpendicular to the current. This phenomenon is utilized in various sensors and devices to measure magnetic field strength.
How does the Quantum Hall Effect differ?
Unlike the classical Hall Effect, the Quantum Hall Effect manifests under extreme conditions of low temperatures and strong magnetic fields, displaying quantized Hall resistance that is used as a resistance standard in metrology.
Why is the Quantum Hall Effect important?
The Quantum Hall Effect is crucial for the precise measurement of fundamental physical constants and serves as a standard for electrical resistance. Its discovery has significant implications for quantum physics, particularly in theories related to the quantum behavior of electrons.
What applications do these effects have?
Both effects are pivotal in sensor technology, with the Hall Effect used in position sensing and the Quantum Hall Effect in defining resistance standards and potentially influencing quantum computing developments.
Conclusion
The exploration of the Hall Effect and Quantum Hall Effect provides not only a cornerstone in the understanding of electromagnetic theory and quantum mechanics but also serves as a foundation for technological advancements. These phenomena have led to the development of critical standards and tools that benefit various scientific fields.
As research continues, the potential for new discoveries and applications inspired by these effects promises to further our capabilities in technology and metrology, reinforcing the relevance of fundamental science in practical innovations.