Physical changes in materials, especially phase transitions like fusion and solidification, play pivotal roles in both the natural world and technological advancements. These processes, fundamental to understanding how substances change states, influence a broad range of phenomena from the formation of natural landscapes to the development of cutting-edge materials. By examining the differences and applications of these transitions, we uncover the intricacies of material behavior and its implications on various scientific fields.
Fusion is the process where a substance transitions from a solid to a liquid state, typically due to an increase in temperature or decrease in pressure. Solidification, conversely, occurs when a liquid material becomes solid, often as a result of cooling or pressure increase. These transformations are crucial in numerous contexts, from geological formations to the manufacturing of metals and alloys, highlighting their importance in both nature and technology.
This article delves into the scientific principles behind fusion and solidification, exploring how these processes impact the natural environment, technological innovations, and research advancements. Through a detailed comparison, we’ll uncover the roles temperature, pressure, and material properties play in determining the behavior of substances undergoing these transformative phase changes.
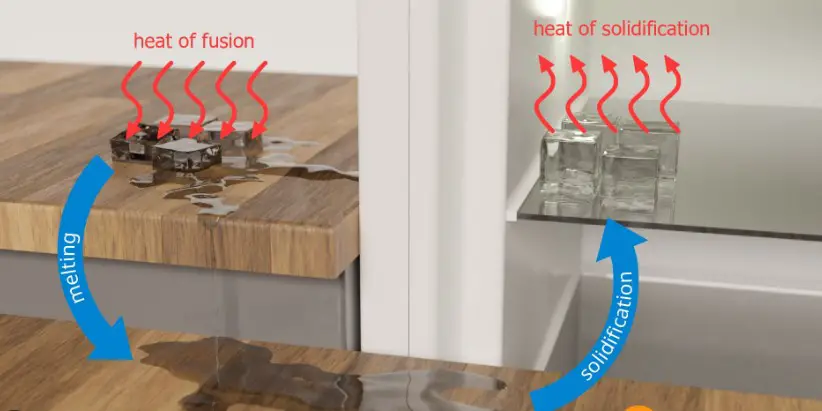
Fusion Explained
Basics of Fusion
Fusion is a physical process where a solid turns into a liquid. This transformation happens when a material reaches its melting point, the temperature at which its solid and liquid phases coexist in equilibrium. At this point, added energy causes the solid particles to vibrate more vigorously until their bonds break, allowing them to move freely and form a liquid.
Fusion is evident in everyday life, from the melting of ice to form water to the more complex procedures used in metalworking where metals are fused to create alloys. These examples underscore fusion’s role in both natural phenomena and human-made processes.
Fusion in Nature
Astronomical Phenomena
In the cosmos, fusion is a cornerstone of stellar life cycles. Stars, including our Sun, are powered by nuclear fusion, a process that combines lighter elements into heavier ones, releasing immense energy. This type of fusion sustains stars and drives the evolution of galaxies, highlighting its fundamental role in the universe.
Biological Processes
On a much smaller scale, fusion occurs in biological systems as well. For example, the fusion of ice in the cryosphere, which includes glaciers and sea ice, plays a crucial role in Earth’s climate system and water cycle, impacting both global temperatures and sea levels.
Fusion in Technology
Nuclear Fusion Energy
Nuclear fusion energy represents the pinnacle of fusion’s application in technology. By mimicking the processes powering the Sun, scientists aim to create a nearly limitless, clean energy source. Despite the technical challenges, such as achieving the extreme temperatures and pressures required, the potential for a sustainable energy solution drives ongoing research and experimentation.
Materials Engineering
In materials engineering, fusion is employed to create new materials with desirable properties. Alloys, created by melting and combining different metals, are a prime example. These materials are crucial in various applications, from construction to electronics, due to their enhanced strength, durability, and resistance to corrosion.
Factors Influencing Fusion
Temperature
The temperature at which fusion occurs is known as the melting point. Each material has a specific melting point that can vary widely, from below zero degrees Celsius for some substances to thousands of degrees for others, like certain metals and alloys.
Pressure
Pressure also plays a significant role in fusion. Increasing pressure generally raises a substance’s melting point by squeezing its particles closer together, making it harder for them to break free and enter the liquid phase.
Material Properties
The chemical composition and structure of a material influence its melting point and the energy required for fusion. For instance, pure substances have a distinct melting point, while mixtures, such as alloys, have a melting range within which they gradually transition from solid to liquid.
Solidification Explained
Basics of Solidification
Solidification, the reverse process of fusion, occurs when a liquid becomes solid. This phase change happens as a material cools below its freezing point, the temperature at which its liquid and solid phases are in equilibrium. During solidification, the motion of particles slows down, allowing forces between them to pull the particles into a fixed, orderly arrangement.
Common examples include the freezing of water into ice and the hardening of lava into volcanic rock. These illustrate how solidification is integral to shaping the natural world and is harnessed in various manufacturing processes.
Solidification in Nature
Geological Formations
The formation of igneous rocks through the cooling and solidification of magma or lava is a key geological process. It creates the diverse landscapes and geological features that characterize the Earth’s crust.
Biological Significance
In the biological realm, solidification is critical in processes like the formation of bone tissue. Minerals solidify within a collagen matrix, giving bones their strength and rigidity, essential for supporting and protecting the body.
Solidification in Manufacturing
Metal Casting and Molding
In manufacturing, solidification is fundamental to metal casting and molding, where molten metal is poured into molds and solidified to form various products. The control of solidification processes is vital to achieving desired product qualities such as shape, texture, and mechanical properties.
3D Printing Technologies
3D printing, or additive manufacturing, often relies on the solidification of materials layer by layer to create objects. This technology uses materials ranging from plastics to metals, exploiting solidification to achieve precision and complexity unattainable with traditional manufacturing methods.
Factors Influencing Solidification
Cooling Rates
The rate at which a material cools can significantly affect the properties of the solidified product. Rapid cooling tends to form finer crystals, increasing the material’s strength, while slow cooling can lead to larger, more defined crystal structures.
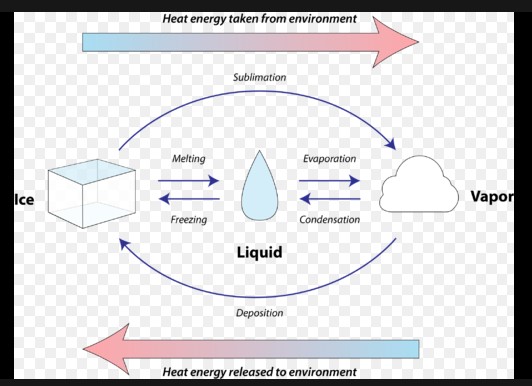
Comparing Fusion and Solidification
Phase Change Processes
Heat Absorption vs. Release
Fusion and solidification are two sides of the same coin, involving heat transfer but in opposite directions. Fusion requires heat absorption to break the bonds holding particles in a solid state, allowing them to move freely as a liquid. Solidification, conversely, involves heat release as particles slow down and bond into a structured solid form. This fundamental difference marks the core of how substances transition between solid and liquid states.
Atomic/Molecular Rearrangements
At the atomic or molecular level, these processes entail significant rearrangement. During fusion, tightly packed particles in a solid start moving apart as they gain energy, becoming less orderly. In solidification, particles in a liquid lose energy, moving closer together and arranging themselves into a more organized structure. These rearrangements are critical for understanding the physical properties of materials in different states.
Application Differences
Industrial Applications
In industry, the practical applications of fusion and solidification vary widely. Fusion processes are crucial in metalworking and glassmaking, where materials must be melted to be shaped or combined with others. Solidification, on the other hand, is foundational in casting and 3D printing, where the controlled cooling of materials is essential for achieving desired shapes and properties.
Scientific Research
From a scientific perspective, studying these phase changes helps in developing new materials and understanding natural phenomena. Researchers explore fusion to understand the behavior of substances under extreme conditions, like those found in space. Solidification studies, meanwhile, contribute to advancements in material sciences, helping to create alloys and composites with tailored properties.
Environmental Impact
Energy Consumption
The energy required for fusion and solidification can be substantial, especially in industrial settings. Fusion processes often demand high temperatures, leading to significant energy consumption. Solidification, while generally requiring less energy directly, still involves cooling systems and other energy-intensive measures to control the process effectively.
Sustainability Considerations
The environmental impact of these processes has led to increased focus on sustainability. Innovations aim to reduce the energy required for melting and cooling and to develop materials that can be recycled or that have a lower environmental footprint during their lifecycle.
Practical Implications
In Material Science
Alloys and Composites
The creation of alloys and composites heavily relies on understanding and controlling fusion and solidification. By manipulating these processes, scientists and engineers can design materials with enhanced strength, corrosion resistance, and other desirable characteristics. This knowledge is crucial for developing advanced materials for use in aerospace, automotive, and construction industries.
Smart Materials Development
Research into phase changes is also driving the development of smart materials. These materials can change their properties in response to external stimuli, such as temperature, light, or magnetic field. The principles of fusion and solidification are integral to designing substances that can transition between different states or configurations, opening up new possibilities in technology and medicine.
In Environmental Science
Climate Change Research
Understanding the fusion and solidification of natural substances is critical for climate change research. The melting of polar ice caps and glaciers involves fusion, while the formation of sea ice is a solidification process. These changes have profound effects on global climate systems, sea levels, and weather patterns, making their study essential for predicting future climate scenarios.
Waste Management Technologies
Solidification is also used in waste management technologies to stabilize hazardous materials, making them safer for disposal or recycling. This application underscores the role of phase change processes in addressing environmental challenges, offering solutions for reducing the impact of human activities on the planet.
In Energy Production
Renewable Energy Sources
The quest for renewable energy sources has sparked interest in harnessing fusion and solidification in innovative ways. Solar thermal power, for example, utilizes the fusion of salts to store heat, which can then be converted into electricity. Similarly, the development of materials for more efficient energy storage often involves precise control over solidification processes.
Efficiency and Safety
Improving efficiency and safety in energy production is another area where understanding these phase changes is vital. In nuclear fusion research, mastering the conditions for controlled fusion reactions could lead to a breakthrough in clean energy. Meanwhile, enhancing the solidification of cooling materials could improve safety and efficiency in nuclear reactors and other energy systems.
FAQs
What triggers fusion in materials?
Fusion in materials is triggered by an increase in temperature or a decrease in pressure, which overcomes the forces holding the particles in a solid state together. This transition to a liquid state is fundamental in various natural and technological processes, including the formation of magma in the Earth’s crust and the melting of metals for casting.
How does solidification affect manufacturing?
Solidification significantly impacts manufacturing, especially in metal casting, plastic molding, and 3D printing. The controlled solidification of materials determines the final product’s structural integrity, mechanical properties, and surface finish, making it crucial for producing high-quality and reliable items.
Can fusion and solidification be reversed?
Yes, fusion and solidification are reversible processes. Fusion occurs when a solid becomes a liquid, and solidification happens when a liquid turns into a solid. These transitions depend on the conditions such as temperature and pressure, allowing for the manipulation of material states in various scientific and industrial applications.
Why are fusion and solidification important in environmental science?
In environmental science, understanding fusion and solidification is essential for studying climate change, water cycle dynamics, and geological phenomena. These processes influence natural events like glacier formation, volcanic activity, and the behavior of polar ice caps, contributing to broader insights into environmental patterns and changes.
Conclusion
The exploration of fusion and solidification unveils the fundamental principles governing the behavior of materials under different conditions. These phase transitions not only provide insights into the natural processes shaping our planet but also underpin technological innovations across diverse industries. Understanding these transformations is crucial for advancing material science, environmental research, and energy production, highlighting the interconnectedness of scientific discovery and practical application.
As we continue to investigate these phenomena, the potential for new materials, improved manufacturing techniques, and sustainable energy solutions becomes increasingly evident. The future of scientific research and technological development relies on our ability to harness and manipulate these processes, emphasizing the importance of continued exploration and understanding of fusion and solidification.