Electron transfer proteins play pivotal roles in various biological processes, including photosynthesis, respiration, and the detoxification of harmful substances. Among these proteins, ferredoxin and rubredoxin are essential for the transfer of electrons in cells, facilitating numerous biochemical reactions. Despite their shared purpose, these proteins exhibit distinct characteristics and functions within different biological systems.
Ferredoxin and rubredoxin are both involved in electron transfer processes but differ in their structure and biological roles. Ferredoxin typically contains iron-sulfur clusters that assist in electron transport within cells, playing a crucial role in photosynthesis and nitrogen fixation. Rubredoxin, on the other hand, usually has a single iron atom bound to its structure and is primarily involved in enzyme catalysis and detoxification processes.
The significance of these proteins extends beyond their immediate functions. By facilitating key biochemical pathways, ferredoxin and rubredoxin contribute to the energy balance within cells and the overall metabolic efficiency of organisms. Their roles in photosynthesis and nitrogen fixation, in particular, underscore their importance in sustaining life on Earth, making them subjects of considerable interest in both scientific research and biotechnological applications.
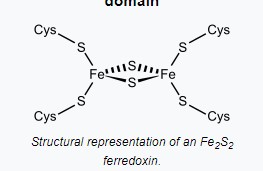
Core Concepts
Electron Transfer Proteins
Definition and Role
Electron transfer proteins are biomolecules that facilitate the transfer of electrons from one molecule to another. This process is critical for the production of energy within cells, playing a central role in metabolic and photosynthetic pathways. Essentially, these proteins are the conduits through which electrons move, driving the biochemical reactions necessary for life.
Ferredoxin Basics
Structural Characteristics
Ferredoxin is characterized by its iron-sulfur (Fe-S) clusters, which are pivotal for its electron transfer capabilities. These clusters are composed of iron and sulfur atoms bonded together and then integrated into the protein structure. This composition allows ferredoxin to efficiently accept and donate electrons, a fundamental aspect of its function in electron transport chains.
Biological Function
The primary role of ferredoxin is to mediate electron transfer in a variety of biochemical pathways, including photosynthesis and nitrogen fixation. In photosynthesis, ferredoxin is involved in the conversion of solar energy into chemical energy, while in nitrogen fixation, it plays a role in the conversion of atmospheric nitrogen into a form that is usable by living organisms. Its ability to participate in these critical processes underscores the importance of ferredoxin in sustaining life.
Rubredoxin Basics
Structural Characteristics
Unlike ferredoxin, rubredoxin contains a single iron atom bound to the protein via cysteine residues, without sulfur atoms making up a cluster. This simpler structure still allows for effective electron transfer, though the mechanism and capacity differ from those of ferredoxin. The single-iron center is crucial for rubredoxin’s role in specific biochemical reactions, particularly those involving enzyme catalysis.
Biological Function
Rubredoxin primarily functions in enzyme catalysis and the detoxification of harmful compounds. Its role in these processes is essential for maintaining cellular health and supporting the metabolism of an organism. By facilitating the transfer of electrons in these contexts, rubredoxin helps to stabilize and activate enzymes necessary for the breakdown of toxic substances and the efficient functioning of metabolic pathways.
Comparative Analysis
Structural Differences
Iron-sulfur Clusters
The presence of iron-sulfur clusters in ferredoxin, as opposed to the single iron atom in rubredoxin, marks a significant structural difference. This variation affects their electron affinity and the types of reactions they can participate in.
Amino Acid Composition
The amino acid composition surrounding the metal sites in these proteins also differs, affecting their stability, reactivity, and interaction with other biomolecules. These differences are key to their distinct functions within cells.
Functional Distinctions
Redox Potential
The redox potential of ferredoxin and rubredoxin varies, influencing their efficiency in electron transfer. Ferredoxin typically operates within a range conducive to its involvement in photosynthesis and nitrogen fixation, while rubredoxin’s redox potential suits its role in enzyme catalysis and detoxification processes.
Biological Roles and Processes
While both proteins are involved in electron transfer, the specific biological processes they participate in differ greatly. Ferredoxin is crucial for energy production and nutrient cycling, whereas rubredoxin’s functions are more focused on cellular maintenance and defense.
Cellular Localization
Distribution in Organisms
Ferredoxin and rubredoxin are found across a wide range of organisms, from bacteria to plants and animals. However, the prevalence and function of these proteins can vary significantly depending on the organism’s specific metabolic needs and environmental conditions.
Intracellular vs. Extracellular
Generally, ferredoxin and rubredoxin are intracellular proteins, but their localization within the cell can differ based on their roles. For example, in plants, ferredoxin is primarily found in the chloroplasts, reflecting its role in photosynthesis.
Role in Photosynthesis
Ferredoxin in Photosynthesis
Electron Transport Chain
In the photosynthetic electron transport chain, ferredoxin acts as a final electron acceptor from the photosystem I complex. It then transfers these electrons to NADP+, reducing it to NADPH, a crucial molecule for the Calvin cycle and the synthesis of glucose.
Interaction with Other Proteins
Ferredoxin’s ability to interact with various proteins, including ferredoxin-NADP+ reductase (FNR), is key to its function in photosynthesis. This interaction facilitates the efficient transfer of electrons and the production of essential molecules for cellular energy.
Rubredoxin’s Role
Lesser-known Functions
Rubredoxin’s involvement in photosynthesis is less direct than that of ferredoxin. Its main roles are related to protective mechanisms against oxidative damage and in the regulation of certain enzymes within the photosynthetic process.
Comparative Analysis with Ferredoxin
While both proteins contribute to the maintenance of the photosynthetic machinery, ferredoxin’s direct role in the electron transport chain contrasts with rubredoxin’s more supportive functions. This distinction highlights the complementary nature of their involvement in photosynthesis and overall cellular health.
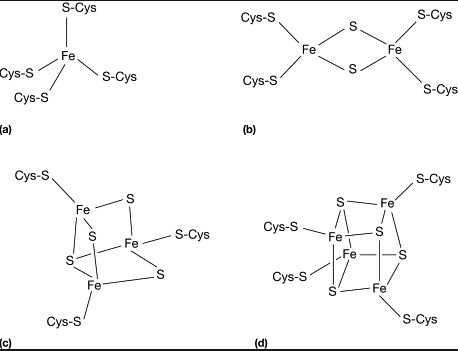
Role in Metabolism
Ferredoxin’s Involvement
Nitrogen Fixation
Ferredoxin is a key player in nitrogen fixation, a process essential for converting atmospheric nitrogen into ammonia, a form that can be used by plants and other organisms. This process occurs in the nitrogenase complex, where ferredoxin donates electrons to nitrogenase, enabling the enzyme to reduce nitrogen gas to ammonia. This reaction is critical for the synthesis of amino acids, nucleic acids, and other nitrogen-containing biomolecules, making ferredoxin indispensable for life on Earth.
Other Metabolic Pathways
Besides nitrogen fixation, ferredoxin participates in various other metabolic pathways, such as the sulfur reduction and carbon fixation pathways. Its role in these pathways further emphasizes its versatility and importance in cellular metabolism, supporting a wide range of biosynthetic reactions and contributing to the energy balance within cells.
Rubredoxin’s Contributions
Detoxification Processes
Rubredoxin plays a crucial role in the detoxification of harmful substances, including reactive oxygen species (ROS) and heavy metals. By facilitating the transfer of electrons to enzymes involved in detoxification processes, rubredoxin helps to protect cells from oxidative damage and maintains cellular integrity and function.
Metal Ion Homeostasis
In addition to its role in detoxification, rubredoxin is involved in metal ion homeostasis, regulating the distribution and availability of metal ions within the cell. This function is vital for the proper activity of metal-dependent enzymes and for protecting the cell from toxic levels of metal ions.
Evolutionary Perspectives
Origins and Evolution
Evolutionary Theories
The evolutionary origins of ferredoxin and rubredoxin trace back to the early stages of life on Earth. Their presence in a wide range of organisms, from bacteria to eukaryotes, suggests that these proteins are among the oldest electron carriers, evolving to support fundamental metabolic processes in diverse living conditions.
Phylogenetic Studies
Phylogenetic studies have provided insights into the evolutionary relationships between ferredoxin, rubredoxin, and other electron transfer proteins. These studies indicate that variations in the structure and function of these proteins have evolved in response to environmental pressures and the metabolic needs of organisms, showcasing the adaptive nature of biological evolution.
Adaptive Significance
Environmental Adaptation
The ability of ferredoxin and rubredoxin to adapt to various environmental conditions has been crucial for the survival and evolution of life. For instance, variations in their structure and function enable organisms to thrive in environments with differing levels of oxygen, light, and nutrients, demonstrating the importance of these proteins in environmental adaptation.
Functional Diversification
Over time, ferredoxin and rubredoxin have undergone functional diversification, acquiring new roles in cellular metabolism and physiology. This diversification has allowed organisms to explore new ecological niches and optimize their metabolic efficiency, further underscoring the evolutionary importance of these proteins.
Technological Applications
Biotechnological Exploits
Bioengineering Prospects
The unique properties of ferredoxin and rubredoxin make them promising tools for bioengineering applications. Scientists are exploring ways to harness these proteins to enhance photosynthesis, improve biofuel production, and develop novel biosensors, leveraging their ability to efficiently transfer electrons.
Industrial Applications
In industrial settings, ferredoxin and rubredoxin are being investigated for their potential to catalyze environmentally friendly reactions. For example, their involvement in electron transfer processes could be exploited in the synthesis of chemicals and pharmaceuticals, minimizing the need for toxic reagents and energy-intensive conditions.
Medical and Environmental
Therapeutic Potentials
The roles of ferredoxin and rubredoxin in cellular metabolism and detoxification have implications for medicine. Researchers are studying these proteins as potential targets for drug development, particularly in the treatment of diseases related to oxidative stress and metabolic disorders.
Bioremediation Efforts
In the environmental arena, the detoxification capabilities of rubredoxin, in particular, offer promising avenues for bioremediation—the use of living organisms to clean up polluted environments. Genetically engineered microbes expressing high levels of rubredoxin could potentially break down toxic compounds more efficiently, offering a sustainable solution to environmental pollution.
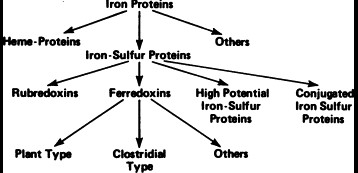
Frequently Asked Questions
What is electron transfer in biology?
Electron transfer in biology refers to the movement of electrons from one molecule to another within biological systems. This process is fundamental to many biochemical reactions, including those involved in energy production, metabolism, and detoxification. Electron transfer proteins, such as ferredoxin and rubredoxin, play critical roles in these reactions by serving as intermediaries that facilitate the transfer of electrons between different molecules.
How do ferredoxin and rubredoxin differ in structure?
The main structural difference between ferredoxin and rubredoxin lies in their metal-binding sites. Ferredoxin typically contains iron-sulfur clusters that participate in electron transfer, whereas rubredoxin possesses a simpler structure with a single iron atom bound by cysteine residues. This structural variance contributes to their distinct biochemical roles and electron transfer capabilities.
Why are ferredoxin and rubredoxin important for life?
Ferredoxin and rubredoxin are crucial for life because they facilitate essential biochemical processes that sustain cellular function and energy production. Ferredoxin is integral to photosynthesis and nitrogen fixation, processes that are vital for plant life and the production of oxygen and usable nitrogen, respectively. Rubredoxin plays a significant role in the detoxification of harmful substances and in various enzyme-catalyzed reactions, helping to maintain cellular health and metabolic balance.
Can ferredoxin and rubredoxin be used in biotechnology?
Yes, ferredoxin and rubredoxin have significant biotechnological applications due to their roles in electron transfer and enzyme catalysis. They are explored for use in bioengineering to enhance photosynthesis, biofuel production, and the bioremediation of polluted environments. Their unique properties make them valuable tools in designing more efficient energy systems and in the development of environmentally friendly technologies.
Conclusion
The exploration of ferredoxin and rubredoxin reveals the complexity and elegance of nature’s mechanisms for sustaining life. These proteins, while serving the common goal of electron transfer, perform their duties in remarkably different ways, reflecting the diversity of life’s strategies for adaptation and survival. Their differences in structure and function highlight the nuanced roles they play in various biological processes, from photosynthesis to detoxification.
Understanding the distinctions between ferredoxin and rubredoxin not only enriches our knowledge of cellular mechanisms but also opens new avenues for scientific discovery and technological innovation. As research continues to uncover the intricate details of these proteins’ actions, their potential to contribute to advancements in energy production, environmental protection, and health care remains vast, underscoring the importance of ongoing exploration in this field.