Substitution reactions stand as pivotal processes in organic chemistry, fundamentally altering molecular structures through the replacement of one atom or group by another. These reactions are integral to the synthesis of a vast array of organic compounds, from pharmaceuticals to polymers, driving advancements in numerous scientific fields. The core of such transformations often involves the interplay between two primary types: electrophilic and nucleophilic substitution.
Electrophilic substitution occurs when an electron-poor atom or group, known as an electrophile, replaces a hydrogen atom in an aromatic or other unsaturated compound. Conversely, nucleophilic substitution involves an electron-rich species, or nucleophile, displacing a leaving group in a saturated or partially saturated molecule. These processes are not only fundamental to organic synthesis but also underpin the structural diversity achievable in organic chemistry.
The distinction between electrophilic and nucleophilic substitution is rooted in the nature of the attacking species and the type of chemical bonds involved. Electrophilic substitutions are characteristic of reactions involving pi bonds and aromatic systems, where electron-rich areas attract electron-poor electrophiles. Nucleophilic substitutions, on the other hand, typically involve sigma bonds, where leaving groups are replaced by nucleophiles, driven by their search for a positive center or an electron-deficient site within a molecule.
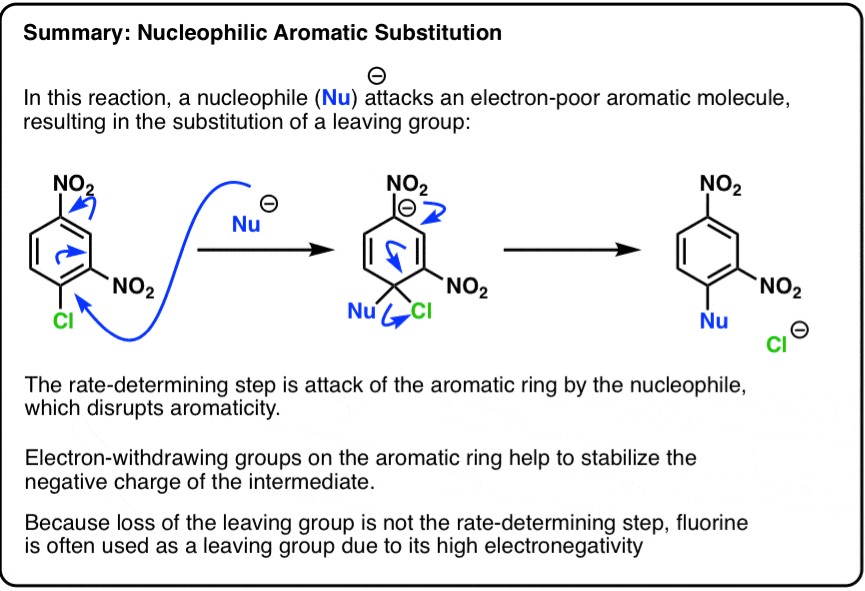
Basic Concepts
Chemical Bonds
Chemical bonds are the glue that holds molecules together. In organic compounds, these bonds form the backbone of the molecule, connecting atoms in a specific arrangement that determines the compound’s properties. There are two main types of chemical bonds relevant to our discussion: covalent and ionic.
- Covalent bonds involve the sharing of electrons between atoms. Most organic compounds are built up of covalent bonds, creating a stable structure that allows for a wide variety of chemical reactions.
- Ionic bonds, on the other hand, involve the transfer of electrons from one atom to another, creating ions that attract each other. While less common in organic chemistry, ionic interactions play a crucial role in the reactivity of some compounds.
Reactivity
The reactivity of a molecule is its tendency to undergo a chemical reaction. Several factors influence this:
- Electronegativity: Atoms with high electronegativity tend to attract electrons more strongly, affecting how molecules interact with each other.
- Bond Strength: Stronger bonds are more difficult to break, influencing how easily a molecule can participate in a reaction.
- Steric Factors: The spatial arrangement of atoms within a molecule can hinder or facilitate reactions, depending on how accessible the reactive sites are.
- Solvent Effects: The medium in which a reaction takes place can significantly impact reactivity, with some solvents stabilizing certain ions or intermediates.
Electrophilic Substitution
Definition
Electrophilic substitution is a reaction where an electrophile replaces a hydrogen atom or another leaving group in a molecule. This type of reaction is characteristic of aromatic compounds, where the rich electron cloud of the benzene ring attracts electrophiles.
Explanation of Electrophiles
Electrophiles are electron-seeking species. They are typically positively charged or neutral atoms lacking in electron density and are attracted to regions of high electron density. In electrophilic substitution reactions, the electrophile attacks an electron-rich site, initiating the substitution process.
Mechanism
The mechanism of electrophilic substitution can be outlined in general steps:
- Formation of the electrophile: The electrophile is generated or activated, making it ready to attack the aromatic ring.
- Attack on the aromatic ring: The electrophile attacks the electron-rich aromatic ring, forming a positively charged intermediate, often referred to as a sigma complex or arenium ion.
- Deprotonation: A base removes a hydrogen ion from the intermediate, restoring the aromaticity and completing the substitution.
Key Features
Electrophilic substitution reactions are marked by several distinct characteristics:
- Preference for aromatic systems: These reactions primarily occur in aromatic compounds due to their stabilized electron-rich aromatic rings.
- Regioselectivity: The position at which the electrophile substitutes the hydrogen atom is often predictable based on the directing effects of substituents already present on the ring.
- Retention of the aromatic system: The aromaticity of the compound is preserved after the reaction, distinguishing it from addition reactions where the aromatic system would be disrupted.
Examples
Common examples of electrophilic substitution reactions include:
- Nitration: The introduction of a nitro group into an aromatic ring using nitric acid and sulfuric acid.
- Sulfonation: The addition of a sulfonic acid group to an aromatic ring, typically using sulfuric acid or sulfur trioxide.
- Friedel-Crafts Alkylation and Acylation: The introduction of alkyl or acyl groups into an aromatic ring, facilitated by aluminum chloride or other Lewis acids.
Nucleophilic Substitution
Definition
Nucleophilic substitution involves the replacement of a leaving group by a nucleophile, an electron-rich species. This reaction is common in aliphatic compounds, where a saturated or partially saturated carbon atom is attached to a leaving group.
Explanation of Nucleophiles
Nucleophiles are species rich in electrons. They seek positively charged or electron-deficient centers to donate their electrons. Nucleophiles can be negatively charged ions (anions) or neutral molecules with lone pairs of electrons.
Mechanism
The general steps involved in nucleophilic substitution include:
- Attack by the nucleophile: The nucleophile attacks the carbon atom bonded to the leaving group, forming a transition state or intermediate.
- Departure of the leaving group: The bond between the carbon atom and the leaving group breaks, completing the substitution.
There are two main mechanisms for nucleophilic substitution: SN1 and SN2, which differ in their steps and conditions.
Key Features
Nucleophilic substitution reactions have several distinct characteristics:
- Involvement of saturated carbons: Unlike electrophilic substitutions that prefer aromatic systems, nucleophilic substitutions often occur at saturated carbon centers.
- Mechanistic pathways: The SN1 and SN2 mechanisms represent two distinct pathways, with differences in their stereochemistry, kinetics, and the effects of solvent and substrate structure.
- Sensitivity to leaving group: The nature of the leaving group significantly influences the reaction rate and mechanism, with better leaving groups facilitating the reaction.
Examples
Common reactions that involve nucleophilic substitution include:
- Halogenation: Replacement of a hydroxyl group with a halogen using hydrohalic acids.
- Dehydration of alcohols: Conversion of alcohols to ethers or alkenes, depending on the conditions.
- Williamson Ether Synthesis: Formation of ethers by reacting an alkoxide with a primary alkyl halide.
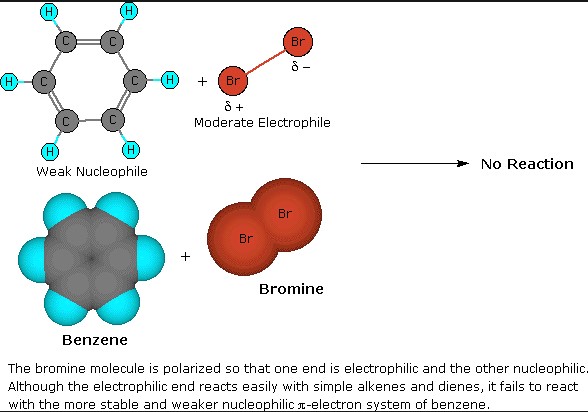
Comparing Mechanisms
Reaction Conditions
Solvents and Temperature
Both electrophilic and nucleophilic substitution reactions are influenced by the choice of solvent and temperature.
- Solvents play a crucial role, especially in nucleophilic substitutions. Polar protic solvents can stabilize ions, favoring SN1 reactions, whereas polar aprotic solvents are better for SN2 reactions as they do not stabilize the nucleophile, allowing for a faster reaction.
- Temperature also affects the speed and outcome. Higher temperatures generally increase reaction rates but can also lead to side reactions or decomposition in sensitive compounds.
Reaction Sites
Site Specificity
The site of reaction in both electrophilic and nucleophilic substitution depends on several factors:
- For electrophilic substitution, the presence of electron-donating or withdrawing groups on the aromatic ring can direct the incoming electrophile to specific positions.
- In nucleophilic substitution, the steric hindrance and the degree of substitution at the carbon atom (primary, secondary, tertiary) play significant roles in determining whether an SN1 or SN2 mechanism will occur.
Reaction Speed
Factors Affecting Speed
The speed of substitution reactions is influenced by the nature of the reactants and the reaction conditions:
- Electrophilic substitution speed depends on the electrophile’s reactivity and the aromatic ring’s electron density.
- For nucleophilic substitution, factors include the nucleophile’s strength, the leaving group’s ability, and the solvent’s properties.
Types of Reactions
SN1 and SN2
Overview and Differences
- SN1 reactions are unimolecular, involving a two-step mechanism with a carbocation intermediate. They are favored by tertiary carbons and polar protic solvents.
- SN2 reactions are bimolecular, involving a single transition state where the nucleophile attacks the substrate as the leaving group departs. SN2 is favored by primary carbons and polar aprotic solvents.
E1 and E2
Overview and Differences
- E1 reactions are elimination reactions that follow a two-step mechanism similar to SN1, leading to the formation of alkenes.
- E2 reactions are also elimination but proceed via a single step, with the base removing a hydrogen atom as the leaving group exits, also resulting in an alkene.
Applications in Organic Synthesis
Pharmaceuticals
Drug Design and Synthesis
Nucleophilic and electrophilic substitution reactions are cornerstone techniques in the design and synthesis of pharmaceuticals. These reactions allow chemists to:
- Modify drug molecules to improve efficacy, reduce side effects, and enhance drug delivery.
- Create complex molecules by systematically building up simpler ones, enabling the discovery of new drugs.
Agrochemicals
Pesticides and Herbicides
Similarly, in the agrochemical industry, these reactions facilitate the synthesis of pesticides and herbicides. Through careful selection of reactants and conditions, chemists can develop compounds that are:
- Selective, targeting specific pests or weeds without harming crops.
- Environmentally safer, with reduced persistence in the ecosystem.
Materials Science
Polymers and Plastics
Substitution reactions also play a vital role in materials science, particularly in the synthesis of polymers and plastics. Through various substitution reactions, polymers can be engineered to have:
- Desired physical properties such as strength, flexibility, and resistance to degradation.
- Functionalities that make them suitable for specific applications, like biodegradable plastics or high-performance fibers.
Challenges and Solutions
Steric Hindrance
Impact on Reaction Pathways
Steric hindrance affects both electrophilic and nucleophilic substitution reactions by:
- Limiting the approach of the electrophile or nucleophile to the reactive site.
- Influencing the choice between SN1 and SN2 mechanisms, where bulky groups favor the SN1 mechanism due to the steric hindrance affecting the SN2 transition state.
Solutions often involve designing the synthesis pathway to minimize steric hindrance or choosing reactants and conditions that mitigate its effects.
Electronic Effects
Influence on Reactivity
Electronic effects, such as the presence of electron-donating or withdrawing groups, significantly impact the reactivity in substitution reactions by:
- Directing the site of electrophilic attack in aromatic compounds.
- Affecting the stability of carbocations in SN1 and the transition states in SN2 mechanisms.
To overcome challenges related to electronic effects, chemists carefully select substituents and reaction conditions to control and direct the outcome of the reaction favorably.
FAQs
What is Electrophilic Substitution?
Electrophilic substitution is a reaction type where an electrophile replaces a hydrogen atom in an aromatic or unsaturated compound. This process is driven by the attraction between the electron-rich aromatic system and the electron-poor electrophile, leading to the formation of new compounds with varied functional groups. It is essential in the synthesis of many organic compounds, including dyes, pharmaceuticals, and agrochemicals.
How Does Nucleophilic Substitution Work?
Nucleophilic substitution involves an electron-rich nucleophile attacking a positively charged or electron-deficient carbon, displacing a leaving group. This mechanism varies, primarily occurring through two pathways: SN1, involving a two-step mechanism with a carbocation intermediate, and SN2, a one-step mechanism where the nucleophile and leaving group simultaneously interact with the carbon atom. This reaction is crucial for creating a wide range of organic compounds.
What Determines Reaction Speed in Substitution Reactions?
The speed of substitution reactions is influenced by several factors, including the nature of the electrophile or nucleophile, the solvent, the leaving group, and the structural features of the substrate. In nucleophilic substitutions, factors like the strength and sterics of the nucleophile and leaving group play significant roles, whereas in electrophilic substitutions, the reactivity of the electrophile and the stability of the intermediate states are crucial.
Why are Substitution Reactions Important in Organic Chemistry?
Substitution reactions are fundamental in organic chemistry because they enable the transformation of simple molecules into complex structures with diverse functional groups. These reactions are instrumental in synthesizing a wide array of organic products, including drugs, agrochemicals, and materials, thereby facilitating advancements in medicine, agriculture, and technology.
Conclusion
Substitution reactions, both electrophilic and nucleophilic, are cornerstone processes in organic chemistry, enabling the transformation and synthesis of complex organic molecules. These reactions not only facilitate the creation of a myriad of compounds with diverse applications but also illustrate the dynamic interplay of electrons within chemical systems. Understanding these processes is essential for advancing in various fields of science and technology, showcasing the power and versatility of organic chemistry.
The distinction between electrophilic and nucleophilic substitution lies at the heart of organic synthesis, guiding chemists in designing and executing reactions that lead to new and useful compounds. As our knowledge and understanding of these reactions continue to grow, so too will our ability to innovate and create, pushing the boundaries of what is possible in organic chemistry and beyond.