Electrochemistry sits at the fascinating intersection of chemistry and electricity, exploring how chemical reactions can create electrical energy and vice versa. It’s a field that has given us batteries, electrolysis, and an understanding of corrosion, among other things. Central to this discipline are two concepts: electrode potential and cell potential. These concepts not only explain the workings of electrochemical cells but also serve as the foundation for designing and optimizing batteries, fuel cells, and corrosion prevention strategies.
Electrode potential refers to the ability of a chemical species to gain or lose electrons, measured under standard conditions against a universal reference. Cell potential, on the other hand, is the measure of the driving force behind the electrical current in an electrochemical cell, representing the difference between the potentials of two electrodes. Together, these potentials determine the direction and magnitude of electrical flow, indicating whether a reaction will occur spontaneously.
Understanding these potentials requires a dive into the principles of electrochemistry, including how they are measured and calculated, and the factors that influence them. Electrode potential and cell potential are crucial for predicting the outcome of electrochemical reactions, designing energy storage devices, and controlling the rate of corrosion in metals. By examining these concepts, we unlock the door to advancements in energy solutions and material science.
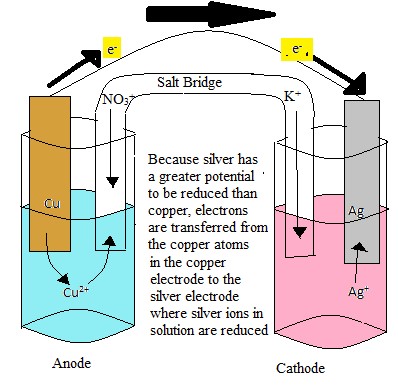
Basics of Electrochemistry
Electrochemistry is a branch of chemistry that studies the relationship between electricity and chemical reactions. This field plays a crucial role in various applications, from batteries and fuel cells to corrosion prevention and electroplating.
Electrode Potential
Definition and Significance
Electrode potential is a measure of the tendency of an electrode to lose or gain electrons when it is in contact with its ion in solution. This property is fundamental in predicting the direction of electron flow in electrochemical cells, influencing the design and efficiency of batteries and other electrochemical devices.
How It Is Measured
The measurement of electrode potential is not straightforward because it involves the comparison of an unknown electrode to a reference. Here’s a simplified step-by-step approach:
- Prepare a standard hydrogen electrode (SHE) as the reference.
- Connect the SHE to the electrode under investigation through a salt bridge.
- Measure the voltage difference using a voltmeter.
This setup allows the electrode potential to be measured accurately, providing insights into the electrochemical behavior of different materials.
Cell Potential
Definition and Significance
Cell potential, also known as electromotive force (EMF), is the voltage or electrical potential difference between two electrodes in an electrochemical cell. It signifies the cell’s ability to drive an electric current through an external circuit, which is key for energy generation and storage devices.
Measuring Cell Potential
Cell potential measurement involves connecting the two electrodes of an electrochemical cell to a voltmeter. The steps include:
- Assemble an electrochemical cell with two electrodes.
- Connect the electrodes to a voltmeter directly.
- Read the voltage, which represents the cell potential.
This process helps determine the efficiency and capacity of electrochemical cells for practical applications.
Key Differences
Nature and Origin
Electrode Potential
Electrode potential is specific to individual half-reactions occurring at the electrode’s surface. It reflects how readily an electrode can gain or lose electrons.
Cell Potential
In contrast, cell potential arises from the complete reaction between two electrodes. It measures the overall ability of the cell to produce or consume electrical energy.
Measurement Techniques
Techniques for Electrode Potential
- Use of a reference electrode: The potential of an electrode is measured against a standard hydrogen electrode or another reference electrode.
- Calibration: Regular calibration of the reference electrode ensures accurate measurements.
Techniques for Cell Potential
- Direct measurement: Cell potential can be measured directly with a voltmeter across the cell’s electrodes.
- Standard conditions: Measurements are often taken under standard conditions to calculate standard cell potential.
Thermodynamics
Role in Determining Spontaneity
- Electrode potential and cell potential play key roles in thermodynamics by determining the spontaneity of reactions. A positive cell potential indicates a spontaneous reaction under standard conditions.
Gibbs Free Energy Relation
- The relationship between cell potential and Gibbs free energy (Δ�ΔG) is given by the equation Δ�=−���ΔG=−nFE, where �n is the number of moles of electrons transferred, �F is Faraday’s constant, and �E is the cell potential. This equation bridges electrochemistry with thermodynamics, providing a quantitative measure of reaction spontaneity.
Factors Influencing Potentials
Electrode Potential Factors
- Ion concentration: Changes in ion concentration can shift electrode potentials due to the Nernst equation.
- Temperature: Increased temperatures can increase electrode potentials by affecting reaction kinetics.
- Metal nature: Different metals have inherent electrode potentials based on their electron affinity and ionization energy.
Cell Potential Factors
- Concentration cells: Variations in ion concentration between two half-cells can affect cell potential.
- Temperature effects: Like electrode potentials, cell potentials are also sensitive to temperature changes.
- Pressure effects: For gases involved in electrochemical reactions, changes in pressure can alter cell potentials.
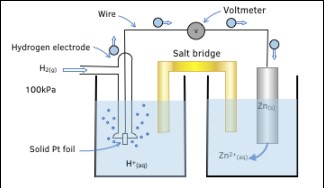
Applications and Examples
Electrochemistry, with its concepts of electrode and cell potential, finds application in a wide array of fields, from industrial processes to everyday devices.
Electrode Potential Applications
Corrosion Prevention
Corrosion, the gradual destruction of metals through chemical reactions with their environment, can be significantly reduced by understanding and manipulating electrode potentials. By applying a protective coating that acts as a more noble metal, we can make the underlying metal act as the cathode, thus preventing its oxidation and corrosion. This method is widely used in galvanic protection techniques.
Metal Plating
Metal plating involves the deposition of a metal coating on an object to enhance its properties, such as resistance to corrosion, appearance, and durability. Electroplating, a key method in metal plating, uses electrode potentials to drive the deposition of metal ions onto an object. By controlling the electrode potential, we can ensure a uniform and high-quality coating.
Cell Potential Applications
Batteries
Batteries, essential for powering countless portable devices, operate on the principle of cell potential. A battery is composed of one or more electrochemical cells, where chemical energy is converted into electrical energy through redox reactions. The difference in electrode potentials between the anode and cathode drives the electrical current, providing power to electronic devices.
Electrolysis Processes
Electrolysis, the process of driving a non-spontaneous chemical reaction through the application of electrical energy, relies heavily on cell potential. In industries, electrolysis is used for the production of chlorine, hydrogen gas, and the purification of metals like aluminum. By manipulating the cell potential, specific reactions can be targeted, optimizing yield and efficiency.
Calculations and Formulas
Standard Electrode Potentials
Calculating Standard Potentials
The standard electrode potential is the voltage difference between a specific electrode and the standard hydrogen electrode under standard conditions. The standard potentials are often found in electrochemical series tables, which list various electrodes and their potential relative to the standard hydrogen electrode.
Nernst Equation for Electrode Potential
The Nernst equation provides a way to calculate the electrode potential under non-standard conditions:
�=�0−����ln�E=E0−nFRTlnQ
- �0E0 is the standard electrode potential.
- �R is the universal gas constant.
- �T is the temperature in Kelvin.
- �n is the number of moles of electrons transferred.
- �F is Faraday’s constant.
- �Q is the reaction quotient.
This equation adjusts the standard potential to reflect changes in concentration, pressure, and temperature.
Standard Cell Potentials
Calculating Cell Potentials
To calculate the standard cell potential, subtract the anode’s (oxidation half-reaction) standard electrode potential from the cathode’s (reduction half-reaction):
�����0=����ℎ���0−������0Ecell0=Ecathode0−Eanode0
This value gives an indication of the cell’s ability to generate an electrical current under standard conditions.
Practical Examples
In practical scenarios, such as in a zinc-copper battery, the standard cell potential can predict the battery’s voltage output. Knowing the standard electrode potentials of zinc and copper, one can easily calculate the cell potential and thus the voltage of the battery.
Challenges and Considerations
Accuracy in Measurements
The accurate measurement of electrode and cell potentials can be challenging due to several factors:
Sources of Error
- Impurities in the electrodes or electrolyte can skew measurements.
- Temperature fluctuations can affect the potentials.
- Junction potentials at the salt bridge can introduce errors.
Overcoming Measurement Challenges
- Purify the reagents and materials to ensure accuracy.
- Conduct measurements in a temperature-controlled environment.
- Use high-quality reference electrodes and maintain them properly.
Real-world Applications
Limitations in Practical Scenarios
In real-world applications, the theoretical potentials might not always match the observed values due to kinetic barriers (like overpotentials), side reactions, or material degradation over time.
Bridging Theory and Application
To effectively bridge the gap between theory and practical application:
- Consider all possible variables that might affect the reaction or device performance.
- Design experiments and devices with a margin for discrepancies.
- Continuously monitor and adjust based on real-world performance data.
FAQs
What is Electrode Potential?
Electrode potential is a measure of the ability of an electrode to either gain electrons and be reduced, or lose electrons and be oxidized. This potential is determined under standard conditions and is compared against a standard hydrogen electrode. It reflects the inherent tendency of a species to acquire electrons and thus participate in electrochemical reactions.
How is Cell Potential Calculated?
Cell potential is calculated by taking the difference between the potentials of the cathode and the anode in an electrochemical cell. This calculation often uses the standard electrode potentials of the electrodes involved, adjusted for the actual reaction conditions using the Nernst equation. The cell potential is a direct indicator of the cell’s ability to produce an electrical current.
Why Are Electrode and Cell Potentials Important?
Electrode and cell potentials are critical for understanding and predicting the spontaneity of electrochemical reactions. They help in the design and optimization of batteries, fuel cells, and electrolysis processes. Moreover, they play a crucial role in preventing corrosion, a common issue in metal objects and structures.
Can Electrode Potential Determine Reaction Direction?
Yes, the electrode potential can help determine the direction of a reaction. A positive electrode potential suggests a spontaneous reaction under standard conditions, indicating that the species is likely to gain electrons (reduction). Conversely, a negative potential suggests that the species is more likely to lose electrons (oxidation).
Conclusion
Electrode and cell potentials are cornerstones of electrochemistry, providing insights into the flow of electrons in chemical reactions and the feasibility of such reactions occurring spontaneously. These concepts not only underpin the science behind batteries and corrosion prevention but also facilitate the development of new technologies for energy storage and conversion. Understanding these potentials allows scientists and engineers to harness electrochemical processes more effectively, paving the way for innovations in sustainable energy solutions.
As we advance in our understanding of electrochemistry, the study of electrode and cell potentials continues to be a critical area of research. With each discovery and technological breakthrough, we edge closer to optimizing energy storage systems and protecting materials from degradation, demonstrating the enduring importance of these fundamental concepts in both scientific exploration and practical application.