The concept of dextrorotatory and levorotatory compounds lies at the heart of organic chemistry, illustrating the fascinating way in which molecules interact with light. These terms describe the unique ability of certain molecules to rotate plane-polarized light either to the right (dextrorotatory) or to the left (levorotatory). This optical activity is not just a curious phenomenon; it’s a critical property that distinguishes molecules with identical compositions but different spatial arrangements.
The difference between dextrorotatory and levorotatory compounds is fundamentally about the direction in which they rotate plane-polarized light. Dextrorotatory compounds rotate light to the right, while levorotatory compounds rotate it to the left. This characteristic is an intrinsic property of chiral molecules, which are non-superimposable on their mirror images, much like left and right hands.
This rotation is not just a theoretical interest but has practical implications across various fields, from pharmaceuticals to food chemistry. The way a molecule rotates light can affect its biological activity, taste, smell, and more. As such, understanding the distinction between dextrorotatory and levorotatory compounds is essential for chemists and industry professionals who seek to harness the unique properties of these molecules for specific applications.
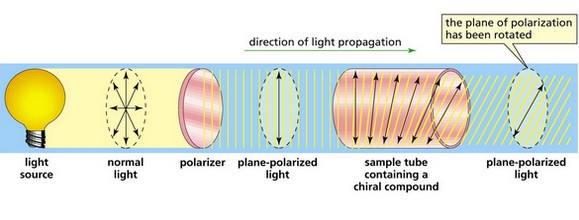
Basic Concepts
Optical Isomerism
Optical isomerism is a type of stereoisomerism where molecules with the same molecular formula and sequence of bonded atoms (constitution) differ only in the three-dimensional orientations of their atoms in space. This difference arises due to the presence of chirality in the molecules, making them non-superimposable on their mirror images—much like left and right hands.
Examples of optical isomerism can be found in many organic compounds, including simple sugars (glucose), amino acids (like alanine), and complex molecules such as ibuprofen. Each of these can exist in two forms that are mirror images of each other, known as enantiomers. For instance, glucose exists as d-glucose and l-glucose, where “d” and “l” indicate the direction in which they rotate plane-polarized light.
Chirality
Chirality refers to a property of asymmetry where an object or a molecule is not superimposable on its mirror image. The term is derived from the Greek word for hand, “cheir,” highlighting the analogy to left and right hands, which are mirror images but cannot be perfectly aligned.
The significance of chirality in chemistry and biology cannot be overstated. It is crucial for understanding how biological molecules recognize and interact with each other. For example, the proteins in our bodies are made of l-amino acids, and DNA helices twist in a right-handed direction. These specific orientations and shapes are vital for the function of biological molecules, affecting how drugs interact with targets in the body or how enzymes catalyze reactions.
Key Differences
Structural Differences
The main structural difference between dextrorotatory and levorotatory compounds lies in their molecular asymmetry. This asymmetry results from the spatial arrangement of atoms around a chiral center, usually a carbon atom bonded to four different groups. These arrangements give rise to two distinct enantiomers for each chiral molecule.
Optical Activity
Optical activity is the ability of chiral molecules to rotate the plane of polarization of plane-polarized light. This rotation can be either to the right, termed dextrorotatory (d- or +), or to the left, termed levorotatory (l- or -). The direction and magnitude of this rotation are specific to each compound and its concentration, as well as the length of the light path through the sample.
Measurement Techniques
Polarimetry is the most common technique used to measure optical activity. It involves shining plane-polarized light through a sample and measuring the angle of rotation with a polarimeter. The steps for using a polarimeter are typically as follows:
- Prepare the sample in a solution or neat (pure) form.
- Fill the polarimeter tube with the sample.
- Shine plane-polarized light through the sample.
- Measure the angle of rotation of the emergent light.
The application of polarimetry extends beyond mere identification of d- or l- enantiomers. It is essential in determining the purity of a substance, the concentration of a solution, and even the rate of a reaction where enantiomers are involved.
Impacts and Applications
Pharmaceutical Industry
In the pharmaceutical industry, the difference between dextrorotatory and levorotatory compounds is critical for drug synthesis and effectiveness. The biological activity of drugs is often dependent on their chirality, with one enantiomer being therapeutically active and the other inactive or even harmful. For instance, the sedative thalidomide has one enantiomer that is effective against morning sickness, while its mirror image caused birth defects. Thus, the synthesis of pure enantiomers and the control of chirality are paramount in drug development.
Food Chemistry
Flavorings and sweeteners are significant applications of chirality in food chemistry. Enantiomers can have different tastes; for example, the d-form of aspartame is sweet, while the l-form is tasteless. This difference means that the optical activity of food additives must be carefully controlled to achieve the desired flavor profiles.
Agricultural Chemistry
In agricultural chemistry, chirality plays a role in the development of pesticides and herbicides. The biological activity of these chemicals can vary dramatically between enantiomers. One might be highly effective at targeting a pest, while the other could be less active or have undesirable environmental effects. The selective synthesis and use of the active enantiomers can lead to more effective and environmentally friendly agricultural practices.
Identifying D and L Isomers
Chemical Methods
Chemical methods offer a traditional but effective approach to distinguishing between D and L isomers. These methods often involve reagents and reactions that are specific to one enantiomer over the other, causing a change that can be easily detected.
- Derivatization: This involves converting the isomers into derivatives that are easier to separate or detect. For example, converting amino acids into their diastereomers using a chiral reagent makes it possible to separate them using standard techniques like chromatography.
- Chiral shift reagents: These are used in NMR spectroscopy to create differences in the shifts of nuclei in D and L isomers, making it easier to distinguish between them.
Physical Methods
Physical methods rely on the inherent physical properties of isomers to distinguish them. Spectroscopy and crystallography are two powerful techniques in this category.
- Circular Dichroism (CD) Spectroscopy: This technique exploits the difference in how D and L isomers absorb left-handed and right-handed circularly polarized light, providing a signature that helps identify the configuration of the molecule.
- X-ray Crystallography: This method can directly reveal the three-dimensional structure of a molecule, including its chirality. By analyzing the arrangement of atoms in a crystal, chemists can determine the absolute configuration of D and L isomers.
Practical Considerations
Synthesis Challenges
The synthesis of pure enantiomers is one of the most significant challenges in chemistry, requiring precise control over the reactions that produce chiral molecules.
- Enantioselective synthesis: This approach involves using catalysts or other means to favor the formation of one enantiomer over the other. Achieving high enantioselectivity is crucial for the economical production of many drugs and chemicals.
- Resolution of racemates: This is a method to separate a mixture of D and L isomers into its components. Techniques like crystallization, chromatography, and kinetic resolution are commonly employed.
Environmental Impact
The environmental impact of chiral molecules, particularly in terms of biodegradation and toxicity, is a growing area of concern.
- Biodegradation: The rate at which chiral pollutants are broken down in the environment can vary significantly between the D and L forms. This difference can affect the persistence and impact of pollutants.
- Toxicity: The toxicity of chiral compounds can also differ between enantiomers. For example, one isomer might be biologically active and toxic, while its mirror image is benign.
Future Directions
Research Trends
Current research trends are focused on overcoming the challenges of chirality through novel synthesis methods and understanding the biological implications of enantiomers.
- Asymmetric organocatalysis: This represents a promising area where small organic molecules are used as catalysts to drive enantioselective reactions.
- Chiral environmental pollutants: Understanding the fate and impact of chiral pollutants in the ecosystem is an area of growing research, aiming to mitigate their effects.
Technological Advancements
Technological advancements are crucial for the improved detection and analysis of chiral molecules, facilitating the development of new drugs and materials.
- Enhanced polarimetry: New polarimeters with higher sensitivity and accuracy allow for the better quantification of optical activity, even in complex mixtures.
- Advanced chromatographic techniques: Developments in chromatography, such as supercritical fluid chromatography (SFC) with chiral columns, have improved the resolution of enantiomers, making it faster and more efficient.
FAQs
How Do You Determine if a Compound is Dextrorotatory or Levorotatory?
The determination of a compound’s optical rotation is typically performed using a polarimeter. By passing plane-polarized light through a sample and observing the direction of rotation, chemists can classify compounds as either dextrorotatory or levorotatory. The sign of the rotation angle indicates the direction: positive for dextrorotatory and negative for levorotatory compounds.
Why is Chirality Important in Pharmaceuticals?
Chirality is crucial in pharmaceuticals because the biological activity of drugs often depends on their three-dimensional shape. Two enantiomers of a chiral drug may have vastly different effects in the body; one might be therapeutic, while the other could be inactive or even harmful. Understanding and controlling chirality is therefore essential for developing safe and effective medications.
Can a Compound Change from Dextrorotatory to Levorotatory?
In general, a compound cannot change from dextrorotatory to levorotatory or vice versa without a chemical change that alters its molecular structure. Such changes might involve the breaking and forming of bonds in a way that affects the molecule’s chirality. Environmental factors alone, such as temperature or pH, typically do not cause such a change in optical activity.
What Role Does Optical Activity Play in Food Chemistry?
Optical activity plays a significant role in food chemistry, particularly in the flavor and aroma of food. Enantiomers can have different tastes and smells; for example, one might be sweet while its mirror image is bitter. Understanding and controlling the optical activity of food ingredients allows food scientists to enhance flavors and create more desirable products.
Conclusion
The exploration of dextrorotatory and levorotatory compounds illuminates the profound impact of molecular structure on chemical behavior and biological activity. These concepts not only enrich our understanding of chemistry but also underscore the importance of chirality in designing substances that benefit our lives, from medicines to food.
As our knowledge of these compounds deepens, so too does our ability to innovate and create more effective, safer products. The study of optical activity, far from being a mere academic curiosity, is a vital tool in the chemist’s arsenal, opening doors to discoveries that can shape the future of multiple industries.